Regional Screening Levels (RSLs) - User's Guide
To download the most recent Regional Screening Level tables, please go to the Generic Tables page. For assistance/questions please use the Regional Screening Levels (RSLs) contact us page.
Disclaimer
This user's guide recommends an approach based upon currently available information about risk assessment on CERCLA sites. This user's guide does not establish binding rules. Alternative approaches for risk assessment may be found to be more appropriate at specific sites, and not every situation is addressed by this guide (e.g., where site circumstances do not match the underlying assumptions, conditions, and models of the user's guide). Decisions to use alternative approaches and a description of any such approach should be documented.
Accordingly, when comments are received at individual CERCLA sites questioning the use of the approaches recommended in this user's guide, the comments should be considered, and an explanation provided for the selected approach.
It should also be noted that the screening levels (SLs) in these tables are based upon human health risk and do not address potential ecological risk. Some sites in sensitive ecological settings may also need to be evaluated for potential ecological risk. EPA's guidance "Ecological Risk Assessment Guidance for Superfund: Process for Designing and Conducting Ecological Risk Assessment" contains an eight step process for using benchmarks for ecological effects in the remedy selection process.
On this page:
1. Introduction
2. Understanding the RSL Website
2.1 General Considerations
2.2 Exposure Assumptions
2.3 Toxicity Values
2.4 Chemical-specific Parameters
2.5 Maximum Contaminant Levels (MCLs)
2.6 Understanding Risk Output on the RSL Website
3. Understanding Your Superfund Site
3.1 Developing a Conceptual Site Model
3.2 Background
3.3 Potential Problems
4. Land Use Descriptions, Equations and Technical Documentation
4.1 Resident
4.2 Composite Worker
4.3 Outdoor Worker
4.4 Indoor Worker
4.5 Construction Worker
4.6 Recreator
4.7 Ingestion of Fish
4.8 Soil to Groundwater
4.9 Supporting Equations and Parameter Discussion
5. Special Considerations
5.1 Cadmium
5.2 Lead
5.3 Manganese
5.4 Vanadium Compounds
5.5 Uranium
5.6 Chromium (VI)
5.7 Aminodinitrotoluenes
5.8 PCBs and Aroclors
5.9 Xylenes
5.10 Arsenic
5.11 Total Petroleum Hydrocarbons (TPHs)
5.12 Soil Saturation Limit (Csat)
5.13 SL Theoretical Ceiling Limit
5.14 Target Risk
5.15 Screening Sites with Multiple Contaminants
5.16 Deriving Soil Gas SLs
5.17 Mutagens
5.18 Trichloroethylene (TCE)
5.19 Mercuric Chloride (and other Mercury salts)
5.20 Cyanide (CN-)
5.21 Thallic Oxide and Thallium Selenite
5.22 Polycyclic Aromatic Hydrocarbon
5.23 Refractory Ceramic Fibers (units in fibers)
5.24 Lanthanum Salts
5.25 MCLs for Trihalomethanes and Haloacetic Acids
5.26 Perchlorate
5.27 Styrene-acrylonitrile trimer (SAN Trimer)
5.28 cis- and trans-Chlordane Surrogate
5.29 Asbestos
5.30 White Phosphorus
6. Recommended Default Exposure Parameters
7. References
1. Introduction
The purpose of this website is to provide default screening tables and a calculator to assist Remedial Project Managers (RPMs), On Scene Coordinators (OSCs), risk assessors, and others involved in decision-making concerning CERCLA hazardous waste sites and to determine whether levels of contamination found at the site may warrant further investigation. Users within and outside the CERCLA program should use the tables or calculator results at their own discretion; they should take care to understand the assumptions incorporated in these results to apply the SLs appropriately.
The SLs presented in the Generic Tables are chemical-specific concentrations for individual contaminants in air, drinking water, and soil that, if exceeded, may warrant further investigation. The SLs generated from the calculator may be default or site-specific concentrations for individual chemicals in soil, air, water, sediment, and fish. Screening levels (SLs) identified on this website are likely to be used early in the process, at Remedial Investigation (RI) scoping and screening of chemicals. In addition, SLs may be initially identified early in the Remedial Investigation/ Feasibility Study (RI/FS) process (for example, at RI scoping) to select appropriate detection limits for RI sampling. It should be emphasized that SLs are not cleanup standards and should not be used as cleanup levels.
The term PRGs (Preliminary Remediation Goals) is used to describe a project team's early and evolving identification of possible remedial goals. PRGs developed in the FS will usually be based on site-specific risks and/or Applicable or Relevant and Appropriate Requirements (ARARs) and not on generic SLs.
2. Understanding the RSL Website
2.1 General Considerations
Risk-based SLs are derived from equations combining exposure assumptions with chemical-specific toxicity values.
2.2 Exposure Assumptions
Generic SLs are based on default exposure parameters and factors that represent Reasonable Maximum Exposure (RME) conditions for long-term/chronic exposures and are based on the methods outlined in EPA's Risk Assessment Guidance for Superfund, Part B Manual (1991) (PDF) (68 pp, 721 K) and Soil Screening Guidance documents (1996 (PDF) (89 pp, 863 K) and 2002 (PDF) (187 pp, 2.2MB).
Site-specific information may warrant modifying the default parameters in the equations and calculating site-specific SLs, which may differ from the values in these tables. In completing such calculations, the user should answer some fundamental questions about the site. For example, information is needed on the contaminants detected at the site, the land use, impacted media and the likely pathways for human exposure.
Whether these generic SLs or site-specific screening levels are used, it is important to clearly demonstrate the equations and exposure parameters used in deriving SLs at a site. A discussion of the assumptions used in the SL calculations should be included in the documentation for a CERCLA site.
2.3 Toxicity Values
In 2003, EPA's Superfund program revised its hierarchy of human health toxicity values, providing three tiers of toxicity values in a memo (PDF) (4 pp, 225 K). Three tier 3 sources were identified in that guidance, but it was acknowledged that additional tier 3 sources may exist. The 2003 guidance did not attempt to rank or put the identified tier 3 sources into a hierarchy of their own. However, when developing the screening tables and calculator presented on this website, EPA needed to establish a hierarchy among the tier 3 sources. The toxicity values used as “defaults” in these tables and calculator are consistent with the 2003 guidance. Chronic and subchronic toxicity values from the following sources, in the order in which they are presented below, are used as the defaults in these tables and calculator.
- EPA's Integrated Risk Information System (IRIS).
- The Provisional Peer Reviewed Toxicity Values (PPRTVs) derived by EPA's Superfund Health Risk Technical Support Center (STSC) for the EPA Superfund program. PPRTVs are archived (removed) when an IRIS profile is released, even if the IRIS profile indicates a toxicity value could not be derived. PPRTVs will retain subchronic values if IRIS releases a profile without subchronic values.
- EPA's Office of Pesticide Programs (OPP) Human Health Benchmarks for Pesticides (HHBPs). In 2016 IRIS archived 51 chemical assessments for pesticides. For these 51 pesticides IRIS has instead recommended the use of the toxicity values presented in the HHBP table. These include RfDs (also referred to as chronic PADs) and OSFs (referred to as cancer quantification values). In 2016, OPP listed 363 pesticides in the HHBP table. Only the 51 archived by IRIS are used in the RSL calculations.
- The Agency for Toxic Substances and Disease Registry (ATSDR) minimal risk levels (MRLs). Current ATSDR toxicity profiles are available here and archived profiles are here. An MRL is an estimate of the daily human exposure to a hazardous substance that is likely to be without appreciable risk of adverse non-cancer health effects over a specified duration of exposure. These substance specific estimates, which are intended to serve as screening levels, are used by ATSDR health assessors and other responders to identify contaminants and potential health effects that may be of concern at hazardous waste sites. ATSDR provides both 'Final' and 'Draft' values, both of which are utilized in the RSLs. Typically, draft values are excluded from the RSL hierarchy. However, ATSDR's draft values have undergone external peer review and meet the criteria for inclusion in the RSL hierarchy. Final values take precedence over draft values in usage, and unique references are provided in the generic tables and calculator output.
- The EPA Office of Water occasionally derives toxicity values presented in Human Health Toxicity Assessments for special case chemicals including PFOA, PFOS, and HFPO-DA.
- The California Environmental Protection Agency Office of Environmental Health Hazard Assessment (OEHHA) provides toxicity values for the State of California. The OEHHA Toxicity Criteria Database website should be monitored for any updates to the toxicity values.
-
In the Fall 2009, this new source of toxicity values used was added: screening toxicity values in an appendix to certain PPRTV assessments. While we have less confidence in a screening toxicity value than in a PPRTV, we put these ahead of HEAST toxicity values because these appendix screening toxicity values are more recent and use current EPA methodologies in the derivation, and because the PPRTV appendix screening toxicity values also receive external peer review. To alert users when these values are used, the key presents an "X" (for Appendix) rather than a "P" (for PPRTV). The following is taken from a PPRTV appendix and states the intended usage of appendix screening levels.
"However, information is available for this chemical, which although insufficient to support derivation of a provisional toxicity value, under current guidelines, may be of limited use to risk assessors. In such cases, the Superfund Health Risk Technical Support Center summarizes available information in an appendix and develops a "screening value." Appendices receive the same level of internal and external scientific peer review as the PPRTV documents to ensure their appropriateness within the limitations detailed in the document. Users of screening toxicity values in an appendix to a PPRTV assessment should understand that there is considerably more uncertainty associated with the derivation of an appendix screening toxicity value than for a value presented in the body of the assessment. Questions or concerns about the appropriate use of screening values should be directed to the Superfund Health Risk Technical Support Center."
- EPA’s HERA program has posted the final Office of Research and Development (ORD) Human Health Toxicity Values for Perfluoropropanoic Acid (PFPrA), Lithium bis[(trifluoromethyl)sulfonyl]azanide (HQ-115), and Trifluoro-N-(trifluoromethanesulfonyl)methanesulfonamide, 1,1,1- (TFSI) . These toxicity assessments provide actionable science to inform EPA decision contexts and were developed in response to a request for site-specific technical support. Please see U.S. EPA. ORD Human Health Toxicity Value for Perfluoropropanoic Acid and U.S. EPA. ORD Human Health Toxicity Value for Lithium bis [(trifluoromethyl)sulfonyl]azanide for more information.
- The 11th Cycle of Groundwater Standards Proposals from the State of Wisconsin Department of Health Services includes recommended standards for 4 PFAS chemicals (Perfluoroundecanoic acid ( PFUDA), Perfluorododecanoic acid (PFDoDA), Perfluorotetradecanoic acid (PFTetDA), and Perfluorooctadecanoic (PFODA)) as well as other chemicals. The EPA Office of Land and Emergency Management’s (OLEM) Human Health Regional Risk Assessment Forum’s (OHHRRAF) Toxicity Workgroup recommends using these values because toxicity values from the other sources do not exist. While the recommended state (Wisconsin) reference values were derived by a state whose toxicity values have not been used before, the state’s toxicity information was determined by the OHHRRAF’s Toxicity Workgroup to be based on similar methods and procedures as those used for other Tier 3 values.
- The EPA Superfund program's Health Effects Assessment Summary Table. Values in HEAST are archived (removed) when an IRIS profile or a PPRTV paper is released, even if the PPRTV paper indicates a toxicity value could not be derived.
Users of these screening tables and calculator wishing to consider using other toxicity values, including toxicity values from additional sources, may find the discussions and seven preferences on selecting toxicity values in the attached Environmental Council of States paper useful for this purpose (ECOS website, ECOS paper(DOC)).
When using toxicity values other than tier 1, users are encouraged to carefully review the basis for the value and to document the basis of toxicity values used on a CERCLA site.
Please contact a Superfund risk assessor in your Region for help with chemicals that lack toxicity values in the sources outlined above.
2.3.1 Reference Doses
The current, or recently completed, EPA toxicity assessments used in these screening tables (IRIS and PPRTVs) define a reference dose, or RfD, as an estimate (with uncertainty spanning perhaps an order of magnitude) of a daily oral exposure to the human population (including sensitive subgroups) that is likely to be without an appreciable risk of deleterious effects during a lifetime. It can be derived from a NOAEL, LOAEL, or benchmark dose, or using categorical regression, with uncertainty factors generally applied to reflect limitations of the data used. RfDs are generally the toxicity value used most often in evaluating noncancer health effects at Superfund sites. Various types of RfDs are available depending on the critical effect (developmental or other) and the length of exposure being evaluated (chronic or subchronic). Some of the SLs in these tables also use Agency for Toxic Substances and Disease Registry (ATSDR) chronic oral minimal risk levels (MRLs) as an oral chronic RfD. Screening toxicity values in an appendix to certain PPRTV assessments were added to the hierarchy in the fall of 2009. The HEAST RfDs used in these SLs were based upon then current EPA toxicity methodologies, but did not use the more recent benchmark dose or categorical regression methodologies. Chronic oral reference doses and ATSDR chronic oral MRLs are expressed in units of (mg/kg-day).
2.3.1.1 Chronic Reference Doses
Chronic oral RfDs are specifically developed to be protective for long-term exposure to a compound. As a guideline for Superfund program risk assessments, chronic oral RfDs generally should be used to evaluate the potential noncarcinogenic effects associated with exposure periods greater than 7 years (approximately 10 percent of a human lifetime). However, this is not a bright line. Note, that ATSDR defines chronic exposure as greater than 1 year for use of their values. The calculator requires the user to select between chronic and subchronic toxicity values.
2.3.1.2 Subchronic Reference Doses
Subchronic oral RfDs are specifically developed to be protective for short-term exposure to a compound. As a guideline for Superfund program risk assessments, subchronic oral RfDs should generally be used to evaluate the potential noncarcinogenic effects of exposure periods between two weeks and seven years. However, this is not a bright line. Note, that ATSDR defines subchronic exposure as less than 1 year for use of their values. The calculator requires the user to select between chronic and subchronic toxicity values.
2.3.2 Reference Concentrations
The current, or recently completed, EPA toxicity assessments used in these screening tables (IRIS and PPRTV assessments) define a reference concentration (RfC) as an estimate (with uncertainty spanning perhaps an order of magnitude) of a continuous inhalation exposure to the human population (including sensitive subgroups) that is likely to be without an appreciable risk of deleterious effects during a lifetime. It can be derived from a NOAEL, LOAEL, or benchmark concentration, or using categorical regression with uncertainty factors generally applied to reflect limitations of the data used. Various types of RfCs are available depending on the critical effect (developmental or other) and the length of exposure being evaluated (chronic or subchronic). These screening tables also use ATSDR chronic inhalation MRLs as a chronic RfC, intermediate inhalation MRLs as a subchronic RfC and California Environmental Protection Agency (chronic) Reference Exposure Levels (RELs) as chronic RfCs. Screening toxicity values in an appendix to certain PPRTV assessments were added to the hierarchy in the fall of 2009. These screening tables may also use some RfCs from EPA's HEAST tables.
2.3.2.1 Chronic Reference Concentrations
The chronic inhalation reference concentration is generally used for continuous or near continuous inhalation exposures that occur for 7 years or more. However, this is not a bright line, and ATSDR chronic MRLs are based on exposures longer than 1 year. EPA chronic inhalation reference concentrations are expressed in units of (mg/m3). Cal EPA RELs are presented in µg/m3 and have been converted to mg/m3 for use in these screening tables. Some ATSDR inhalation MRLs are derived in parts per million (ppm) and some in mg/m3. For use in this table all were converted into mg/m3. The calculator requires the user to select between chronic and subchronic toxicity values.
2.3.2.2 Subchronic reference Concentrations
The subchronic inhalation reference concentration is generally used for exposures that are between 2 weeks and 7 years. However, this is not a bright line, and ATSDR subchronic MRLs are based on exposures less than 1 year. EPA subchronic inhalation reference concentrations are expressed in units of (mg/m3). Cal EPA RELs are presented in µg/m3 and have been converted to mg/m3 for use in these screening tables. Some ATSDR intermediate inhalation MRLs are derived in parts per million (ppm) and some in mg/m3. For use in this table all were converted into mg/m3. The calculator requires the user to select between chronic and subchronic toxicity values.
2.3.3 Slope Factors
A slope factor and the accompanying weight-of-evidence determination are the toxicity data most commonly used to evaluate potential human carcinogenic risks. Generally, the slope factor is a plausible upper-bound estimate of the probability of a response per unit intake of a chemical over a lifetime. The slope factor is used in risk assessments to estimate an upper-bound lifetime probability of an individual developing cancer as a result of exposure to a particular level of a potential carcinogen. Slope factors should always be accompanied by the weight-of-evidence classification to indicate the strength of the evidence that the agent is a human carcinogen.
Oral slope factors are toxicity values for evaluating the probability of an individual developing cancer from oral exposure to contaminant levels over a lifetime. Oral slope factors are expressed in units of (mg/kg-day)-1. When available, oral slope factors from EPA's IRIS or PPRTV assessments are used. The ATSDR does not derive cancer toxicity values (e.g. slope factors or inhalation unit risks). Some oral slope factors used in these screening tables were derived by the California Environmental Protection Agency, whose methodologies are quite similar to those used by EPA's IRIS and PPRTV assessments. Screening toxicity values in an appendix to certain PPRTV assessments were added to the hierarchy in the fall of 2009. When oral slope factors are not available in IRIS then PPRTVs, Cal EPA assessments, PPRTV appendices or values from HEAST are used.
2.3.4 Inhalation Unit Risk
The IUR is defined as the upper-bound excess lifetime cancer risk estimated to result from continuous exposure to an agent at a concentration of 1 µg/m3 in air. Inhalation unit risk toxicity values are expressed in units of (µg/m3)-1.
When available, inhalation unit risk values from EPA's IRIS or PPRTV assessments are used. The ATSDR does not derive cancer toxicity values (e.g. slope factors or inhalation unit risks). Some inhalation unit risk values used in these screening tables were derived by the California Environmental Protection Agency, whose methodologies are quite similar to those used by EPA's IRIS and PPRTV assessments. Screening toxicity values in an appendix to certain PPRTV assessments were added to the hierarchy in the fall of 2009. When inhalation unit risk values are not available in IRIS then PPRTVs, Cal EPA assessments, PPRTV appendices or values from HEAST are used.
2.3.5 Toxicity Equivalence Factors (TEF)
Some chemicals are members of the same family and exhibit similar toxicological properties; however, they differ in the degree of toxicity. Therefore, it is recommended that a toxicity equivalence factor (TEF) be applied to the measured concentrations in environmental media to create a toxicity equivalent concentration. In the case of dioxins and furans, the toxicity equivalent concentrations are summed and compared to the 2,3,7,8-TCDD RSL. In the case of the numbered PCBs, the TEF has been applied to the toxicity value, and the measured concentration in environmental media can be compared directly to the RSLs for the individual numbered PCBs.
The following table contains the various dioxin-like toxicity equivalency factors for Dioxins, Furans and dioxin-like PCBs (Van den Berg et al. 2006 (PDF) (19 pp, 290 K)), which are the World Health Organization 2005 values. These TEFs are also presented in the May 2013 fact sheet, " Use of Dioxin TEFs in Calculating Dioxin TEQs at CERCLA and RCRA Sites (PDF)" (8 pp, 105 K) which references the 2010 EPA report, "Recommended Toxicity Equivalence Factors (TEFs) for Human Health Risk Assessments of 2,3,7,8Tetrachlorodibenzo-p-dioxin and Dioxin-Like Compounds (PDF)" (38 pp, 532 K).
Dioxin Toxicity Equivalence Factors
CASRN | Dioxins and Furans | TEF | |
---|---|---|---|
Chlorinated dibenzo-p-dioxins
|
|||
1746-01-6 | 2,3,7,8-TCDD | 1 | |
40321-76-4 | 1,2,3,7,8-PeCDD | 1 | |
39227-28-6 | 1,2,3,4,7,8-HxCDD | 0.1 | |
57653-85-7 | 1,2,3,6,7,8-HxCDD | 0.1 | |
19408-74-3 | 1,2,3,7,8,9-HxCDD | 0.1 | |
35822-46-9 | 1,2,3,4,6,7,8-HpCDD | 0.01 | |
3268-87-9 | OCDD | 0.0003 | |
Chlorinated dibenzofurans
|
|||
51207-31-9 | 2,3,7,8-TCDF | 0.1 | |
57117-41-6 | 1,2,3,7,8-PeCDF | 0.03 | |
57117-31-4 | 2,3,4,7,8-PeCDF | 0.3 | |
70648-26-9 | 1,2,3,4,7,8-HxCDF | 0.1 | |
57117-44-9 | 1,2,3,6,7,8-HxCDF | 0.1 | |
72918-21-9 | 1,2,3,7,8,9-HxCDF | 0.1 | |
60851-34-5 | 2,3,4,6,7,8-HxCDF | 0.1 | |
67562-39-4 | 1,2,3,4,6,7,8-HpCDF | 0.01 | |
55673-89-7 | 1,2,3,4,7,8,9-HpCDF | 0.01 | |
39001-02-0 | OCDF | 0.0003 | |
PCBs
|
|||
IUPAC No. | Structure | ||
Non-ortho
|
|||
32598-13-3 | 77 | 3,3',4,4'-TetraCB | 0.0001 |
70362-50-4 | 81 | 3,4,4',5-TetraCB | 0.0003 |
57465-28-8 | 126 | 3,3',4,4',5-PeCB | 0.1 |
32774-16-6 | 169 | 3,3',4,4',5,5'-HxCB | 0.03 |
Mono-ortho
|
|||
32598-14-4 | 105 | 2,3,3',4,4'-PeCB | 0.00003 |
74472-37-0 | 114 | 2,3,4,4',5-PeCB | 0.00003 |
31508-00-6 | 118 | 2,3',4,4',5-PeCB | 0.00003 |
65510-44-3 | 123 | 2',3,4,4',5-PeCB | 0.00003 |
38380-08-4 | 156 | 2,3,3',4,4',5-HxCB | 0.00003 |
69782-90-7 | 157 | 2,3,3',4,4',5'-HxCB | 0.00003 |
52663-72-6 | 167 | 2,3',4,4',5,5'-HxCB | 0.00003 |
39635-31-9 | 189 | 2,3,3',4,4',5,5'-HpCB | 0.00003 |
Di-ortho*
|
|||
35065-30-6 | 170 | 2,2',3,3',4,4',5-HpCB | 0.0001 |
35065-29-3 | 180 | 2,2',3,4,4',5,5'-HpCB | 0.00001 |
* Di-ortho values come from Ahlborg, U.G., et al. (1994), which are the WHO 1994 values from Toxic equivalency factors for dioxin-like PCBs: Report on WHO-ECEH and IPCS consultation, December 1993 Chemosphere, Volume 28, Issue 6, March 1994, Pages 1049-1067 (PDF) (19 pp, 880 K).
2.3.6 Relative Potency Factors (RPFs)
Some chemicals are members of the same family and exhibit similar toxicological properties; however, they differ in the degree of toxicity. Therefore, a relative potency factor (RPF) must first be applied to adjust the oral slope factor or inhalation unit risk based on the relative potency to the primary compound. In the case of the PAHs, the RPF has been applied to the toxicity value, and the measured concentration in environmental media can be compared directly to the RSLs for the individual PAHs.
Carcinogenic polycyclic aromatic hydrocarbons
Provisional Guidance for Quantitative Risk Assessment of Polycyclic Aromatic Hydrocarbons (EPA/600/R-93/089, July 1993), recommends that a RPF be used to convert concentrations of carcinogenic polycyclic aromatic hydrocarbons (cPAHs) to an equivalent concentration of benzo(a)pyrene when assessing the cancer risks posed by these substances from oral exposures. These RPFs are based on the potency of each compound relative to that of benzo(a)pyrene. For the toxicity value database, these RPFs have been applied to the toxicity values. Although this is not in complete agreement with the direction in the aforementioned documents, this approach was used so that toxicity values could be generated for each cPAH. Additionally, it should be noted that computationally it makes little difference whether the RPFs are applied to the concentrations of cPAHs found in environmental samples or to the toxicity values as long as the RPFs are not applied to both. However, if the adjusted toxicity values are used, the user will need to sum the risks from all cPAHs as part of the risk assessment to derive a total risk from all cPAHs. A total risk from all cPAHs is what is derived when the RPFs are applied to the environmental concentrations of cPAHs and not to the toxicity values. These RPFs are not needed, and should not be used, with the Cal EPA toxicity values, nor should they be used when calculating non-cancer risk.
The IRIS Profile gives the following instructions for RPF application:
"It (BaP) also serves as an index chemical for deriving relative potency factors to estimate the carcinogenicity of other PAH congeners, such as in EPA's Relative Potency Factor approach for the assessment of the carcinogenicity of PAHs (U.S. EPA, 1993) (PDF) (28 pp, 1.4 MB)."
and
"The inhalation unit risk for benzo[a]pyrene is derived with the intention that it will be paired with EPA's relative potency factors for the assessment of the carcinogenicity of PAH mixtures. In addition, regarding the assessment of early life exposures, because cancer risk values calculated for benzo[a]pyrene were derived from adult animal exposures, and because benzo[a]pyrene carcinogenicity occurs via a mutagenic mode of action, exposures that occur during development should include the application of ADAFs..."
The following table presents the RPFs for cPAHs recommended in Provisional Guidance for Quantitative Risk Assessment of Polycyclic Aromatic Hydrocarbons (PDF) (28 pp, 1.4 MB).
Relative Potency Factors for Carcinogenic Polycyclic Aromatic Hydrocarbons
CASRN | Compound | RPF |
---|---|---|
50-32-8 | Benzo(a)pyrene | 1.0 |
56-55-3 | Benz(a)anthracene | 0.1 |
205-99-2 | Benzo(b)fluoranthene | 0.1 |
207-08-9 | Benzo(k)fluoranthene | 0.01 |
218-01-9 | Chrysene | 0.001 |
53-70-3 | Dibenz(a,h)anthracene | 1.0 |
193-39-5 | Indeno(1,2,3-c,d)pyrene | 0.1 |
2.4 Chemical-specific Parameters
Several chemical specific parameters are needed for development of the RSLs.
2.4.1 Sources
Many sources are used to populate the database of chemical-specific parameters. Major sources are curated as individual data tables and designated by the name of the source (i.e.; PHYSPROP, EPI, CRC, etc.). Minor sources are curated together in an individual data table designated as "Other". The sources are briefly described below.
The major sources are:
-
The Physical Properties Database (PHYSPROP) was developed by Syracuse Research Corporation (SRC). The PhysProp database contains chemical structures, names and physical properties for over 41,000 chemicals. Physical properties collected from a wide variety of sources include experimental, extrapolated and estimated values.
-
The Estimation Programs Interface (EPI SuiteTM) was developed by the US Environmental Protection Agency's Office of Pollution Prevention and Toxics and SRC. These programs estimate various chemical-specific properties. The calculations for these SL tables use the experimental values for a property over the estimated values.
-
CRC Handbook of Chemistry and Physics. (Various Editions)
-
Perry's Chemical Engineers' Handbook (Various Editions).McGraw-Hill. Online version available here. Green, Don W.; Perry, Robert H. (2008).
-
Lange's Handbook of Chemistry (Various Editions). Online version available here. Speight, James G. (2005). McGraw-Hill.
-
Yaws' Handbook of Thermodynamic and Physical Properties of Chemical Compounds. Knovel, 2003.
-
EPA Supplemental Guidance for Developing Soil Screening Levels for Superfund Sites (SSL) and Appendix A-C (39 pp, 681 K), "Chemical Properties and Regulatory/Human Health Benchmarks for SSL Calculations". Table C-1: Chemical-Specific Properties used in SSL Calculations and Table C-4: Metal Kd Values (L/kg) as a Function of pH.
-
Summary of Physical/Chemical and Environmental Parameters for PFAS : Subject to Interim Special Order by Consent No. 20-086-CWP/AP/GW/HW/DW/SW, paragraph 37(J)(3). Environmental Studies Report E21-0037. 3M, 2021. Data from the March 2000 report, Sulfonated Perfluorochemicals in the Environment: Sources, Dispersion, Fate, and Effects, is also used.
-
Baes, C.F. 1984. Oak Ridge National Laboratory. A Review and Analysis of Parameters for Assessing Transport of Environmentally Released Radionuclides through Agriculture. Values are also found in Superfund Chemical Data Matrix (SCDM).
-
U.S. EPA 2004. Risk Assessment Guidance for Superfund Volume I: Human Health Evaluation Manual (Part E, Supplemental Guidance for Dermal Risk Assessment) Final. OSWER 9285.7-02EP. July 2004. Document (PDF) (186 pp, 4.2 MB) and website.
-
U.S. EPA 2015 Technical Guide for Assessing and Mitigating the Vapor Intrusion Pathway from Subsurface Vapor Sources to Indoor Air. OSWER Publication 9200.2-154 and associated calculation spreadsheet (VISL).
Some of the more commonly used minor sources are:
-
The PubChem website published by the National Center for Biotechnology Information, U.S. National Library of Medicine, 8600 Rockville Pike, Bethesda, MD20894, USA.
-
The Hazardous Substance Data Bank (HSDB) website published by the U.S. National Library of Medicine 8600 Rockville Pike, Bethesda, MD 20894 National Institutes of Health, Health & Human Services.
-
CompTox Chemicals Dashboard website published by the US Environmental Protection Agency containing measured and predicted data for 883,000 chemicals.
-
The Agency for Toxic Substances & Disease Registry (ATSDR) Toxicological Profiles. Agency for Toxic Substances and Disease Registry, 4770 Buford Hwy NE, Atlanta, GA 30341.
-
NIOSH Pocket Guide to Chemical Hazards (NPG), NIOSH Publication No. 97-140, February 2004.
-
WATER9 Version 2.0 is the Windows-based wastewater treatment model containing a database listing many organic compounds and procedures for obtaining reports of constituent fates, including air emissions and treatment effectiveness. This program supersedes WATER8, Chem9, and Chemdat8 WATER9.
-
CHEMFATE Database. CHEMFATE is part of the Environmental Fate Data Bases (EFDB) software developed by SRC under sponsorship of the U.S. Environmental Protection Agency. CHEMFATE contains physical property values, rate constants, and monitoring data for approximately 1700 chemicals.
-
The ARS Pesticide Properties Database: U.S. Department of Agriculture, Agricultural Research Service. 2009. Document (PDF) (393 pp, 775 K) and website.
-
Other sources for esoteric chemicals with sparsely published physicochemical parameters.
2.4.2 Hierarchy by Parameter
The hierarchies below present the major sources in order of selection. Minor sources are interspersed throughout the hierarchies in a collection called "Other". These hierarchies are generally representative of both organic and inorganic compounds. The 3M chemical properties for PFAS is the first source for all parameters for PFAS compounds.
- Organic Carbon Partition Coefficient (Koc) (L/kg). Not applicable for inorganics. Other; EPI estimated values; SSL, Yaws' estimated values; EPI experimental values; Yaws' Experimental values. The exception to this hierarchy are the nine ionizable organics identified in table 42 of Part 5 of the Soil Screening Guidance Technical Background Document (PDF) (28 pp, 523 K). Appendix L goes into detail on the derivation of these values. The table is reproduced below:
Compound Koc
pH=6.8FBenzoic acid 0.6 2-chlorophenol 388 2,4-dichlorophenol 147 2,4-dinitrophenol 0.01 pentachlorophenol (PCP) 592 2,3,4,5-tetrachlorophenol 4742 2,3,4,6-tetrachlorophenol 280 2,4,5-trichlorophenol 1597 2,4,6-trichlorophenol 381 -
Dermal Permeability Coefficient (Kp) (cm/hour). EPI estimated values; RAGS Part E estimated value.
-
Effective Predictive Domain (EPD). Calculated based on RAGS Part E criteria for MW and log Kow.
-
Fraction Absorbed (FA). RAGS Part E Exhibit B-3; Calculated. Calculated FA values less than zero are set to zero.
-
Molecular Weight (MW) (g/mole). PHYSPROP; EPI; CRC; Perry's; Lange's; Yaws'; Other.
-
Water Solubility (S) (mg/L at 25 °C, unless otherwise stated in the source). PHYSPROP experimental values; EPI experimental values; CRC; Yaws' experimental values; Perry's; Lange's; PHYSPROP estimated values; Yaws estimated values; EPI estimated values (WATERNT v.1.01, WSKOWWIN v1.42 respectively); Other.
-
Unitless Henry's Law Constant (H' at 25 °C, unless otherwise stated in the source.). PHYSPROP experimental values; EPI experimental values; Yaws' experimental values; CRC; PHYSPROP extrapolated values; PHYSPROP estimated values; EPI group-estimated values; EPI bond-estimated values; Other.
-
Henry's Law Constant (atm-m3/mole at 25 °C, unless otherwise stated in the source). PHYSPROP experimental values; EPI experimental values; Yaws' experimental values; CRC; PHYSPROP extrapolated values; PHYSPROP estimated values; EPI group-estimated values; EPI bond-estimated values; Other.
-
Diffusivity in Air (Dia) (cm2/s). WATER9 equations.
-
Diffusivity in Water (Diw) (cm2/s). WATER9 equations.
-
Fish Bioconcentration Factor (BCF) (L/kg). EPI experimental values; EPI estimated values; Other.
-
Soil-Water Partition Coefficient (Kd) (cm3/g). For inorganics: SSL; BAES; Other. For organics the Kd = foc × Koc except for PFAS compounds where a Kd may come from the 3M Environmental Studies Report.
-
Density (g/cm3). CRC; Perry's; Lange's; Yaws'; Other.
-
Melting Point (MP °C). PHYSPROP experimental values; EPI experimental values; CRC; Perry's; Lange's; Yaws' freezing point; EPI estimated values; Other.
-
log Octanol-Water Partition Coefficient (logKow). PHYSPROP experimental values, EPI experimental values; CRC; Yaws experimental values; PHYSPROP extrapolated; PHYSPROP estimated; EPI estimated values; Yaws estimated values; Other.
-
Vapor Pressure (VP). PHYSPROP experimental values, EPI experimental values; CRC; PHYSPROP extrapolated values; PHYSPROP estimated values; EPI estimated values; Other.
-
Critical Temperature (Tc °K). CRC; Yaws' Experimental; Yaws' Estimated; VISL; Other.
-
Enthalpy of vaporization at the normal boiling point (cal/mol). CRC; VISL; Yaws' extrapolated; Yaws' Estimated.
-
Lower Explosive Limit (LEL). CRC; Yaws' experimental; Yaws' extrapolated; Yaws' estimated; VISL
2.5 Maximum Contaminant Levels (MCLs)
The Safe Drinking Water Act (SDWA) was originally passed by Congress in 1974 to protect public health by regulating the nation's public drinking water supply. SDWA authorizes the United States Environmental Protection Agency (US EPA) to set national health based standards for drinking water to protect against both naturally-occurring and man-made contaminants that may be found in drinking water.
US EPA sets national standards for drinking water based on sound science to protect against health risks, considering available technology and costs. These National Primary Drinking Water Regulations set enforceable maximum contaminant levels (MCLs) for contaminants in drinking water or required ways to treat water to remove contaminants. More information can be found in the 2018 Drinking Water Standards and Advisory Tables. The standards that are set to “treat water to remove contaminants” are Maximum Residual Disinfectant Levels (MRDLs). MRDLs are a specific type of MCL that are equally enforceable. They are presented together in the RLS tables and calculator output under the general term of MCL. The contaminants with MRDLs are: monochloramine, dichloramine, trichloramine, organic chloramine, chlorine, and chlorine dioxide. In 2024, EPA finalized MCLs for six PFAS in drinking water. PFOA, PFOS, PFHxS, PFNA, and HFPO-DA as contaminants with individual MCLs, and PFAS mixtures containing at least two or more of PFHxS, PFNA, HFPO-DA, and PFBS using a Hazard Index MCL to account for the combined and co-occurring levels of these PFAS in drinking water.
US EPA sets primary drinking water standards through a three-step process: First, US EPA identifies contaminants that may adversely affect public health and occur in drinking water with a frequency and at levels that pose a threat to public health. Second, US EPA determines a maximum contaminant level goal (MCLG) for contaminants it decides to regulate. This goal is the level of a contaminant in drinking water below which there is no known or expected risk to health. Third, US EPA specifies a MCL, the maximum permissible level of a contaminant in drinking water which is delivered to any user of a public water system. These levels are enforceable standards, and are set as close to the MCLGs as feasible.
MCLs are provided in the RSL tables and the calculator output for users’ information. A few things should be understood about the differences between RSLs and MCLs.
- RSLs are generated by and for the Superfund program, and MCLs are generated by the Office of Water although they are both used by other federal and state programs.
- The RSL calculations may result in a lower concentration than the MCL for a contaminant. The most common reason for this is that the RSLs represent risk-based concentrations considering only the relationship between exposure and risk.
- RSLs are calculated considering ingestion, inhalation, and dermal exposure to tap water.
- MCLs are set as close to risk-based goals, or Maximum Contaminant Level Goal (MCLG), as feasible. MCLGs are non-enforceable public health goals. MCLGs consider only public health and not the limits of detection and treatment technology effectiveness. Conversely, MCLs take the best available analytical and treatment technologies and cost into consideration.
- MCLGs for noncancer effects are based on a Reference Dose and consider ingestion of drinking water with a relative source contribution. The relative source contribution is the percentage of total drinking water exposure for the general population, after considering other exposure routes (for example, food, inhalation).
- For chemical contaminants that are carcinogens, EPA sets the MCLG at zero if: 1) there is evidence that a chemical may cause cancer and 2) there is no dose below which the chemical is considered safe. If a chemical is carcinogenic, and a safe dose can be determined, EPA sets the MCLG at a level above zero that is safe.
- If you have questions about the use of MCLs and/or RSLs at a Superfund site, consult your regional risk assessor.
2.6 Understanding Risk Output on the RSL Website
The RSL calculator provides an option to select risk output. In the calculator, select yes if risk output is desired. Selecting risk output requires the calculator to be run in "Site-Specific" mode. In site-specific mode, the user will be required to enter site concentrations for each media and chemical selected. The "Soil to Groundwater" medium does not have risk output and the risk option will become disabled when selected. The risk and hazard index values presented on this site are chemical-specific values for individual contaminants in air, water, soil, and fish that may warrant further investigation or site cleanup.
This portion of the risk assessment process is generally referred to as "Risk Characterization". This step incorporates the outcome of the exposure and toxicity assessments to calculate the risk resulting from potential exposure to chemicals via the pathways and routes of exposure determined appropriate for the source area.
2.6.1 How Risk is Calculated
The process used to calculate risk (carcinogenic risk and hazard quotient) in this calculator does not follow the traditional method of first calculating a Chronic Daily Intake (CDI). Rather, risk is derived using a simple method that relies on the linear nature of the relationship between concentration and risk. Using the equation below, an RSL, the target risk or target hazard quotient used to calculate the RSL, and a concentration entered by the user are all that is required to calculate risk.
Carcinogenic: TR / RSL = Risk / C
Noncarcinogenic: THQ / RSL = HQ / C
The linear equation above is then rearranged to solve for risk:
Carcinogenic: Risk = (C × TR) / RSL
Noncarcinogenic: HQ = (C × THQ) / RSL
where:
Risk = a unitless probability of an individual developing cancer over a lifetime, determined with the equation above;
HQ = unitless ratio of exposure concentration to reference concentration where a value less than or equal to unity indicates the exposure is not likely to result in adverse health effects.;
C = Concentration entered by the user in site-specific mode [mg/kg ; μg/m3 ; μg/L];
TR = Target Risk provided by the user in site-specific mode;
THQ = Target Hazard Quotient provided by the user in site-specific mode;
RSL = Regional Screening Level, determined by the values entered by the user in site-specific mode [mg/kg ; μg/m3 ; μg/L].
2.6.2 One-Hit Rule for Carcinogenic Risk
The linear risk equation, listed above, is valid only at low risk levels (below estimated risks of 0.01). For sites where chemical intakes might be high (estimated risks above 0.01, an alternate calculation should be used. The one-hit equation, which is consistent with the linear low-dose model, should be used instead (RAGS Part A, ch. 8). The results presented use this rule. In the following instances, the one-hit rule is used independently in the risk output tables:
- Risk from a single exposure route for a single chemical.
- Summation of single chemical risk (without one-hit rule applied to single chemical results) for multiple exposure routes (right of each row).
- Summation of risk (without one-hit rule applied to single chemical results) from a single exposure route for multiple chemicals (bottom of each column).
- Summation of total risk (without one-hit rule applied to single chemical results or summations listed above) from multiple chemicals across multiple exposure routes (bottom right hand cell).
3. Understanding Your Superfund Site
The "Generic Tables" page provides generic concentrations in the absence of site-specific exposure assessments. These concentrations can be used for:
-
Prioritizing multiple sites or operable units or areas of concern within a facility or exposure units.
-
Setting risk-based detection limits for contaminants of potential concern (COPCs).
-
Focusing future site investigation and risk assessment efforts (e.g., selecting COPCs for the baseline risk assessment).
-
Identifying contamination which may warrant cleanup.
-
Identifying sites, or portions of sites, which warrant no further action or investigation.
-
Initial cleanup goals when site-specific data are lacking.
Generic SLs are provided for multiple exposure pathways and for chemicals with both carcinogenic and noncarcinogenic effects. The most protective of the carcinogenic and noncarcinogenic values is selected as the RSL. Two sets of summary tables are provided. Both consider a 10-6 target cancer risk level; however, one set considers a target hazard quotient of 1.0 and the other considers a target hazard quotient of 0.1.The summary tables identify whether the SL is based on cancer or noncancer effects by including a "c" or "n" after the SL. Site-specific SLs corresponding to an HQ of 0.1 may be appropriate for those sites where multiple chemicals are present that have RfDs or RfCs based on the same toxic endpoint.
Consideration of cumulative noncancer hazard and cumulative cancer risk is important when using the RSLs on a site-specific basis (refer to Section 5.14 and Section 5.15). In general, the site-specific Hazard Index for chemicals with RfDs or RfCs based on the same toxic endpoint should not exceed a value of 1.0 (calculated by summing the Hazard Quotients for individual chemicals). Use of the noncancer RSLs based on a target, Hazard Quotient of 0.1 is recommended for initial screening of sites where more than one chemical with the same toxic endpoint might be present.
The use of RSLs for carcinogens based on a target risk of 10-6 for general site screening purposes is normally adequate to address cumulative risk. Site-specific SLs based upon a cancer risk greater than 10-6 can be calculated and may be appropriate based upon site-specific considerations. However, caution is recommended to ensure that cumulative cancer risk for all actual and potential carcinogenic contaminants found at the site does not have a residual (after site cleanup, or when it has been determined that no site cleanup is required) cancer risk exceeding 10-4. Also, changing the target risk or HI may change the balance between the cancer and noncancer endpoints. At some concentrations, the cancer-risk concerns predominate; at other concentrations, noncancer-HI concerns predominate. The user must take care to consider both when adjusting target risks and hazards.
Tables are provided in either MS Excel or in PDF format. The following lists the tables provided and a description of what is contained in each:
-
Summary Table - provides a list of contaminants, toxicity values, MCLs and the lesser (more protective) of the cancer and noncancer SLs for resident soil, industrial soil, resident air, industrial air and tapwater.
-
Residential Soil Supporting Table - provides a list of contaminants, toxicity values and the cancer and noncancer SLs for resident soil.
-
Industrial Soil Supporting Table - provides a list of contaminants, toxicity values and the cancer and noncancer SLs for industrial soil.
-
Residential Air Supporting Table - provides a list of contaminants, toxicity values and the cancer and noncancer SLs for resident air.
-
Industrial Air Supporting Table - provides a list of contaminants, toxicity values and the cancer and noncancer SLs for industrial air.
-
Residential Tapwater Supporting Table - provides a list of contaminants, toxicity values, MCLs and the cancer and noncancer SLs for tapwater.
3.1 Developing a Conceptual Site Model
When using generic SLs at a site, the exposure pathways of concern and site conditions should match those used in developing the SLs presented here. (Note, however, that future uses may not match current uses. Future uses are potential site uses that may occur in the future. At Superfund sites, future uses should be considered as well as current uses. RAGS Part A, Chapter 6, provides guidance on selecting future-use receptors.) Thus, it is necessary to develop a conceptual site model (CSM) to identify likely contaminant source areas, exposure pathways, and potential receptors. This information can be used to determine the applicability of SLs at the site and the need for additional information. The final CSM diagram represents linkages among contaminant sources, release mechanisms, exposure pathways, and routes and receptors based on historical information. It summarizes the understanding of the contamination problem. A separate CSM for ecological receptors can be useful. Part 2 and Attachment A of the Soil Screening Guidance for Superfund: Users Guide (EPA 1996) contains the steps for developing a CSM.
As a final check, the CSM should address the following questions:
-
Are there potential ecological concerns?
-
Is there potential for land use other than those used in the SL calculations (i.e., residential and commercial/industrial)?
-
Are there other likely human exposure pathways that were not considered in development of the SLs?
-
Are there unusual site conditions (e.g. large areas of contamination, high fugitive dust levels, potential for indoor air contamination)?
The SLs and later PRGs may need to be adjusted to reflect the answers to these questions.
Below is a potential CSM of the quantified pathways addressed in the SL Tables.
3.2 Background
EPA may be concerned with two types of background at sites: naturally occurring and anthropogenic. Natural background is usually limited to metals whereas anthropogenic (i.e. human-made) “background” includes both organic and inorganic contaminants.
Please note that the SL tables, which are purely risk-based, may yield SLs lower than naturally occurring background concentrations of some chemicals in some areas. However, background considerations may be incorporated into the assessment and investigation of sites, as acknowledged in existing EPA guidance. Background levels should be addressed as they are for other contaminants at CERCLA sites. For further information see EPA's guidance Role of Background in the CERCLA Cleanup Program (PDF) (13 pp, 144 K), April 2002, (OSWER 9285.6-07P) and Guidance for Comparing Background and Chemical Concentration in Soil for CERCLA Sites (PDF) (89 pp, 1.2 MB), September 2002, (OSWER 9285.7-41).
Generally EPA does not clean up below natural background. In some cases, the predictive risk-based models generate SL concentrations that lie within or even below typical background concentrations for the same element or compound. Arsenic, aluminum, iron and manganese are common elements in soils that have background levels that may exceed risk-based SLs. This does not mean that these metals cannot be site-related, or that these metals should automatically be attributed to background. Attribution of chemicals to background is a site-specific decision; consult your regional risk assessor.
Where anthropogenic “background” levels exceed SLs and EPA has determined that a response action is necessary and feasible, EPA's goal will be to develop a comprehensive response to the widespread contamination. This will often require coordination with different authorities that have jurisdiction over the sources of contamination in the area.
3.3 Potential Problems
As with any risk based screening table or tool, the potential exists for misapplication. In most cases, this results from not understanding the intended use of the SLs or PRGs. In order to prevent misuse of the SLs, the following should be avoided:
- Applying SLs to a site without adequately developing a conceptual site model that identifies relevant exposure pathways and exposure scenarios.
- Not considering the effects from the presence of multiple contaminants, where appropriate.
- Use of the SLs as cleanup levels without adequate consideration of the other NCP remedy selection criteria on CERCLA sites.
- Use of SL as cleanup levels without verifying numbers with a toxicologist or regional risk assessor.
- Use of outdated SLs when tables have been superseded by more recent values.
- Not considering the effects of additivity when screening multiple chemicals.
- Applying inappropriate target risks or changing a cancer target risk without considering its effect on noncancer, or vice versa.
- Not performing additional screening for pathways not included in these SLs (e.g,. vapor intrusion, fish consumption).
- Adjusting SLs upward by factors of 10 or 100 without consulting a toxicologist or regional risk assessor.
4. Land Use Descriptions, Equations and Technical Documentation
The SLs consider human exposure to individual contaminants in air, drinking water and soil. The equations and technical discussion are aimed at developing risk-based SLs or PRGs. The following text presents the land use equations and their exposure routes. Table 1 presents the definitions of the variables and their default values. Any alternative values or assumptions used in developing SLs on a site should be presented with supporting rationale in the decision document on CERCLA sites.
4.1 Resident
4.1.1 Resident Soil
This receptor spends most, if not all, of the day at home. The activities for this receptor involve typical home making chores (cooking, cleaning and laundering) as well as outdoor activities. The resident is assumed to be exposed to contaminants via the following pathways: incidental ingestion of soil, dermal contact with soil, inhalation of volatiles and fugitive dust. Adults and children exhibit different ingestion rates for soil. For example, the child resident is assumed to ingest 200 mg per day while the adult ingests 100 mg per day. To account for changes in intake as the receptor ages, age adjusted intake equations were developed.
Note that the soil ingestion rates are intended to also represent ingestion of indoor dust. According to U.S. EPA 2011, “The source of the soil in these recommendations could be outdoor soil, indoor containerized soil used to support growth of indoor plants, or a combination of both outdoor soil and containerized indoor soil. The inhalation and subsequent swallowing of soil particles is accounted for in these recommended values, therefore, this pathway does not need to be considered separately.” Further, according to U.S. EPA 1997, “Although the recommendations presented below are derived from studies which were mostly conducted in the summer, exposure during the winter months when the ground is frozen or snow covered should not be considered as zero. Exposure during these months, although lower than in the summer months, would not be zero because some portion of the house dust comes from outdoor soil.”
This land use is for developing residential default screening levels that are presented in the RSL Generic Tables.
4.1.1.1 Noncarcinogenic-child
The residential soil land use equation, presented here, contains the following exposure routes:
- incidental ingestion of soil
- dermal contact with soil
- inhalation of volatiles and particulates emitted from soil
- Total
4.1.1.2 Noncarcinogenic-adult
The residential soil land use equation, presented here, contains the following exposure routes:
- incidental ingestion of soil
- dermal contact with soil
- inhalation of volatiles and particulates emitted from soil
- Total
4.1.1.3 Carcinogenic
The residential soil land use equation, presented here, contains the following exposure routes:
- incidental ingestion of soil
- dermal contact with soil
- inhalation of volatiles and particulates emitted from soil
- Total
4.1.1.4 Mutagenic
The residential soil land use equation, presented here, contains the following exposure routes:
- incidental ingestion of soil
- dermal contact with soil
- inhalation of volatiles and particulates emitted from soil
- Total
4.1.1.5 Vinyl Chloride - Carcinogenic
The residential soil land use equations, presented here, contain the following exposure routes:
- incidental ingestion of soil
- dermal contact with soil
- inhalation of volatiles and particulates emitted from soil
- Total
4.1.1.6 Trichloroethylene - Carcinogenic and Mutagenic
The residential soil land use equations, presented here, contain the following exposure routes:
- incidental ingestion of soil
- dermal contact with soil
- inhalation of volatiles and particulates emitted from soil
- Total.
A number of studies have shown that inadvertent ingestion of soil is common among children 6 years old and younger (Calabrese et al. 1989, Davis et al. 1990, Van Wijnen et al. 1990). Therefore, the dose method uses an age-adjusted soil ingestion factor that takes into account the difference in daily soil ingestion rates, body weights, and exposure duration for children from 1 to 6 years old and others from 7 to 26 years old. The equation is presented below. This health-protective approach is chosen to take into account the higher daily rates of soil ingestion in children as well as the longer duration of exposure that is anticipated for a long-term resident. For more on this method, see RAGS Part B.
4.1.1.7 Supporting Equations
- Child
- Adult
- Age-adjusted
4.1.2 Resident Tapwater
This receptor is exposed to chemicals in water that are delivered into a residence from sources such as groundwater or surface water. Ingestion of drinking water is an appropriate pathway for all chemicals. The inhalation exposure route is only calculated for volatile compounds. Activities such as showering, laundering, and dish washing contribute to contaminants in the air for inhalation. Dermal contact with tapwater is also considered for analytes determined to be within the effective predictive domain as described in Section 4.9.8.
This land use is for developing residential default screening levels that are presented in the RSL Generic Tables.
4.1.2.1 Noncarcinogenic-child
The tapwater land use equation, presented here, contains the following exposure routes:
- ingestion of water
- dermal
- inhalation of volatiles
- Total
4.1.2.2 Noncarcinogenic-adult
The tapwater land use equation, presented here, contains the following exposure routes:
- ingestion of water
- dermal
- inhalation of volatiles
- Total
4.1.2.3 Carcinogenic
The tapwater land use equation, presented here, contains the following exposure routes:
- ingestion of water
- dermal
- inhalation of volatiles
- Total
4.1.2.4 Mutagenic
The tapwater land use equation, presented here, contains the following exposure routes:
- ingestion of water
- dermal
- inhalation of volatiles
- Total
4.1.2.5 Vinyl Chloride - Carcinogenic
The tapwater land use equation, presented here, contains the following exposure routes:
- ingestion of water
- dermal
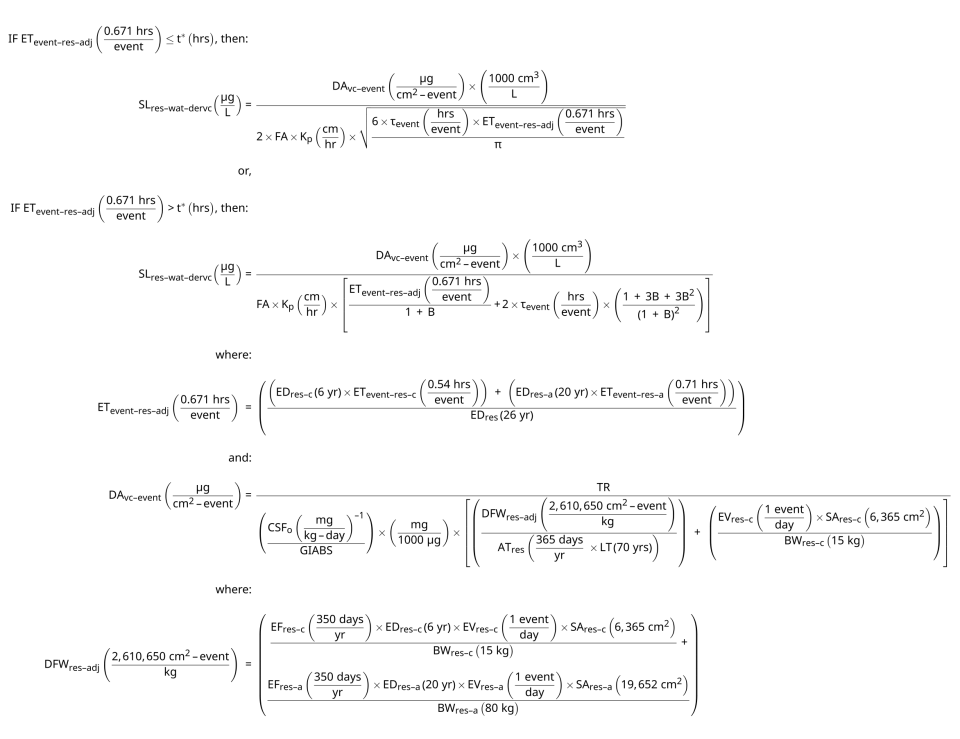
- inhalation of volatiles
- Total
4.1.2.6 Trichloroethylene - Carcinogenic and Mutagenic
The tapwater land use equation, presented here, contains the following exposure routes:
- ingestion of water
- dermal
- inhalation of volatiles
- Total
4.1.2.7 Supporting Equations
- Child
- Adult
- Age-adjusted
4.1.3 Resident Air
This receptor spends most, if not all, of the day at home. The activities for this receptor involve typical home making chores (cooking, cleaning and laundering) as well as outdoor activities. The resident is assumed to be exposed to contaminants via the following pathway: inhalation of ambient air. This land use has no assumptions of how contaminants get into the air and the RSLs derived should be compared to air samples.
This land use is for developing residential default screening levels that are presented in the RSL Generic Tables.
4.1.3.1 Noncarcinogenic
The air land use equation, presented here, contains the following exposure routes:
- inhalation
4.1.3.2 Carcinogenic
The air land use equation, presented here, contains the following exposure routes:
- inhalation
4.1.3.3 Mutagenic
The air land use equation, presented here, contains the following exposure routes:
- inhalation
4.1.3.4 Vinyl Chloride - Carcinogenic
The air land use equation, presented here, contains the following exposure routes:
- inhalation
4.1.3.5 Trichloroethylene - Carcinogenic and Mutagenic
The air land use equation, presented here, contains the following exposure routes:
- inhalation
4.2 Composite Worker
4.2.1 Composite Worker Soil
This is a long-term receptor exposed during the work day who is a full-time employee working on-site and spends most of the workday conducting maintenance activities outdoors. The activities for this receptor (e.g., moderate digging, landscaping) typically involve on-site exposure to surface soils. The composite worker is expected to have an elevated soil ingestion rate (100 mg per day) and is assumed to be exposed to contaminants via the following pathways: incidental ingestion of soil, dermal contact with soil, inhalation of volatiles and fugitive dust. The composite worker combines the most protective exposure assumptions of the outdoor and indoor workers. The only difference between the outdoor worker and the composite worker is that the composite worker uses the more protective exposure frequency of 250 days/year from the indoor worker scenario.
This land use is for developing industrial default screening levels that are presented in the RSL Generic Tables.
4.2.1.1 Noncarcinogenic
The composite worker soil land use equation, presented here, contains the following exposure routes:
- incidental ingestion of soil
- dermal exposure
- inhalation of volatiles and particulates emitted from soil
- Total
4.2.1.2 Carcinogenic
The composite worker soil land use equation, presented here, contains the following exposure routes:
- incidental ingestion of soil
- dermal exposure
- inhalation of volatiles and particulates emitted from soil
- Total
4.2.2 Composite Worker Air
This is a long-term receptor exposed during the work day who is a full-time employee working on-site and spends most of the workday conducting maintenance activities indoors. The composite worker is assumed to be exposed to contaminants via the following pathway: inhalation of ambient air. The composite worker combines the most protective exposure assumptions of the outdoor and indoor workers. The only difference between the outdoor worker and the composite worker is that the composite worker uses the more protective exposure frequency of 250 days/year from the indoor worker scenario. This land use has no assumptions of how contaminants get into the air and the RSLs derived should be compared to air samples.
This land use is for developing industrial default screening levels that are presented in the RSL Generic Tables.
4.2.2.1 Noncarcinogenic
The air land use equation, presented here, contains the following exposure routes:
- Inhalation
4.2.2.2 Carcinogenic
The air land use equation, presented here, contains the following exposure routes:
- Inhalation
4.3 Outdoor Worker
4.3.1 Outdoor Worker Soil
This is a long-term receptor exposed during the work day who is a full-time employee working on-site and spends most of the workday conducting maintenance activities outdoors. The activities for this receptor (e.g., moderate digging, landscaping) typically involve on-site exposure to surface soils. The outdoor worker is expected to have an elevated soil ingestion rate (100 mg per day) and is assumed to be exposed to contaminants via the following pathways: incidental ingestion of soil, dermal contact with soil, inhalation of volatiles and fugitive dust. The outdoor worker receives more exposure than the indoor worker under commercial/industrial conditions.
The outdoor worker soil land use is not provided in the RSL Generic Tables but RSLs can be created by using the Calculator.
4.3.1.1 Noncarcinogenic
The outdoor worker soil land use equation, presented here, contains the following exposure routes:
- incidental ingestion of soil
- dermal exposure
- inhalation of volatiles and particulates emitted from soil
- Total
4.3.1.2 Carcinogenic
The outdoor worker soil land use equation, presented here, contains the following exposure routes:
- incidental ingestion of soil,
- dermal exposure,
- inhalation of volatiles and particulates emitted from soil,
- Total.
4.3.2 Outdoor Worker Air
This is a long-term receptor exposed during the work day who is a full-time employee working on-site and spends most of the workday conducting maintenance activities outdoors. The outdoor worker is assumed to be exposed to contaminants via the following pathway: inhalation of ambient air. This land use has no assumptions of how contaminants get into the air and the RSLs derived should be compared to air samples.
The outdoor worker air land use is not provided in the RSL Generic Tables but RSLs can be created by using the Calculator.
4.3.2.1 Noncarcinogenic
The air land use equation, presented here, contains the following exposure routes:
- Inhalation

4.3.2.2 Carcinogenic
The air land use equation, presented here, contains the following exposure routes:
- Inhalation

4.4 Indoor Worker
4.4.1 Indoor Worker Soil
This receptor spends most, if not all, of the workday indoors. Thus, an indoor worker has no direct dermal contact with outdoor soils. This worker may, however, be exposed to contaminants through ingestion of contaminated soils that have been incorporated into indoor dust and inhalation of volatiles and particulates from outside soils. RSLs calculated for this receptor are expected to be protective of both workers engaged in low intensity activities such as office work and those engaged in more strenuous activity (e.g., factory or warehouse workers).
The indoor worker soil land use is not provided in the Generic Tables but RSLs can be created by using the Calculator.
4.4.1.1 Noncarcinogenic
The indoor worker soil land use equation, presented here, contains the following exposure routes:
- incidental ingestion of soil
- inhalation of volatiles and particulates emitted from soil
- Total
4.4.1.2 Carcinogenic
The indoor worker soil land use equation, presented here, contains the following exposure routes:
- incidental ingestion of soil
- inhalation of volatiles and particulates emitted from soil
- Total
4.4.2 Indoor Worker Air
This is a long-term receptor exposed during the work day who is a full-time employee working on-site and spends most of the workday conducting maintenance activities indoors. The indoor worker is assumed to be exposed to contaminants via the following pathway: inhalation of ambient air. This land use has no assumptions of how contaminants get into the air and the RSLs derived should be compared to air samples.
The indoor worker air land use is not provided in the Generic Tables but RSLs can be created by using the Calculator.
4.4.2.1 Noncarcinogenic
The air land use equation, presented here, contains the following exposure routes:
- Inhalation
4.4.2.2 Carcinogenic
The air land use equation, presented here, contains the following exposure routes:
- Inhalation

4.5 Construction Worker
An assessment for the construction worker scenario is described in more detail in the Supplemental Soil Screening Guidance (SSSG, EPA, 2002). Despite the exposure duration of one year, carcinogenic risk is averaged over an assumed lifetime of 70 years, consistent with the assumption that the risk of developing cancer continues even after exposure has stopped. EPA guidance states that the averaging time for noncancer is to be set at the same length as exposure duration, even if the exposure duration is less than one year. For noncancer, the averaging time can be changed to be less than a year by changing the number of weeks worked (EW). Further, the examples given in the SSSG show that the time of traffic (Tt) is equivalent to EF and time of construction (Tc) is the averaging time (length of project).
The particulate emission factor (PEF) and volatilization factor (VF) equations used are unique to this scenario. See Section 4.9 for further information on subchronic VFs and PEFs. The PEFs calculated in these scenarios may predict much higher air concentrations than the standard wind-driven PEFs; however, the inhalation screening level will likely be dominated by the VF in the case of a volatile contaminant. VFs are commonly 5 orders of magnitude more protective than PEFs. Additionally, the ingestion route typically is the driving factor in most RSL calculations. Two types of mechanical soil disturbance are addressed: standard vehicle traffic (unpaved) and other construction activities (wind, grading, dozing, tilling and excavating). In general, the intake and contact rates are all greater than the outdoor worker. Exhibit 5-1 in the supplemental soil screening guidance presents the exposure parameters
The construction worker soil land use is not provided in the Generic Tables but RSLs can be created by using the Calculator.
4.5.1 Construction Worker Soil Exposure to Standard Vehicle Traffic
This is a short-term receptor exposed during the work day working around vehicles suspending dust in the air. The activities for this receptor (e.g., trenching, excavating) typically involve on-site exposure to surface soils. The construction worker is expected to have an elevated soil ingestion rate (330 mg per day) and is assumed to be exposed to contaminants via the following pathways: incidental ingestion of soil, dermal contact with contaminants in soil, inhalation of volatiles and fugitive dust. The only difference between this construction worker and the one described in section 4.5.2 is that this construction worker uses a different PEF. The construction worker soil land use is not provided in the Generic Tables but RSLs can be created by using the Calculator. The construction land use is described in the supplemental soil screening guidance. This land use is limited to an exposure duration of 1 year and is thus, subchronic. Other unique aspects of this scenario are that the PEF is based on mechanical disturbance of the soil. Two types of mechanical soil disturbance are addressed: standard vehicle traffic and other than standard vehicle traffic (e.g. wind, grading, dozing, tilling and excavating). In general, the intakes and contact rates are all greater than the outdoor worker. Exhibit 5-1 in the supplemental soil screening guidance presents the exposure parameters.
4.5.1.1 Noncarcinogenic
The construction worker soil land use equation, presented here, contains the following exposure routes:
- incidental ingestion of soil
- dermal exposure
- inhalation of volatiles and particulates emitted from soil
- Total
4.5.1.2 Carcinogenic
The construction worker soil land use equation, presented here, contains the following exposure routes:
- incidental ingestion of soil,
- dermal exposure,
- inhalation of volatiles and particulates emitted from soil,
- Total.
4.5.2 Construction Worker Soil Exposure to Other Construction Activities
This is a short-term receptor exposed during the work day working around heavy vehicles suspending dust in the air. The activities for this receptor (e.g., dozing, grading, tilling, dumping, and excavating) typically involve on-site exposure to surface soils. The construction worker is expected to have an elevated soil ingestion rate (330 mg per day) and is assumed to be exposed to contaminants via the following pathways: incidental ingestion of soil, dermal contact with contaminants in soil, inhalation of volatiles and fugitive dust. The only difference between this construction worker and the one described in section 4.5.1 is that this construction worker uses a different PEF. The construction worker soil land use is not provided in the Generic Tables but RSLs can be created by using the Calculator. The construction land use is described in the supplemental soil screening guidance. This land use is limited to an exposure duration of 1 year and is thus, subchronic. Other unique aspects of this scenario are that the PEF is based on mechanical disturbance of the soil. Two types of mechanical soil disturbance are addressed: standard vehicle traffic and other than standard vehicle traffic (e.g. wind, grading, dozing, tilling and excavating). In general, the intakes and contact rates are all greater than the outdoor worker. Exhibit 5-1 in the supplemental soil screening guidance presents the exposure parameters.
4.5.2.1 Noncarcinogenic
The construction worker soil land use equation, presented here, contains the following exposure routes:
- incidental ingestion of soil
- dermal exposure
- inhalation of volatiles and particulates emitted from soil
- Total
4.5.2.2 Carcinogenic
The construction worker soil land use equation, presented here, contains the following exposure routes:
- incidental ingestion of soil
- dermal exposure
- inhalation of volatiles and particulates emitted from soil
- Total
4.6 Recreator
4.6.1 Recreator Soil or Sediment
This receptor spends time outside involved in recreational activities. The recreator is assumed to be exposed to contaminants via the following pathways: incidental ingestion of soil, dermal contact with contaminants in soil, and inhalation of volatiles and fugitive dust. There are no default RSLs for this scenario; only site-specific.
The recreator soil land use is not provided in the Generic Tables but RSLs can be created by using the Calculator.
4.6.1.1 Noncarcinogenic - Child
The recreator soil or sediment land use equation, presented here, contains the following exposure routes:
- incidental ingestion of soil or sediment
- dermal contact with soil or sediment
- inhalation of volatiles and particulates emitted from soil or sediment
- Total
4.6.1.2 Noncarcinogenic - Adult
The recreator soil or sediment land use equation, presented here, contains the following exposure routes:
- incidental ingestion of soil or sediment
- dermal contact with soil or sediment
- inhalation of volatiles and particulates emitted from soil or sediment
- Total
4.6.1.3 Carcinogenic
The recreator soil or sediment land use equation, presented here, contains the following exposure routes:
- incidental ingestion of soil or sediment
- dermal contact with soil or sediment
- inhalation of volatiles and particulates emitted from soil or sediment
- Total
4.6.1.4 Mutagenic
The recreator soil or sediment land use equation, presented here, contains the following exposure routes:
- incidental ingestion of soil or sediment,
- dermal contact with soil or sediment
- inhalation of volatiles and particulates emitted from soil or sediment
- Total
4.6.1.5 Vinyl Chloride - Carcinogenic
The recreator soil or sediment land use equations, presented here, contain the following exposure routes:
- incidental ingestion of soil or sediment
- dermal contact with soil or sediment
- inhalation of volatiles and particulates emitted from soil or sediment
- Total
4.6.1.6 Trichloroethylene - Carcinogenic and Mutagenic
The recreator soil or sediment land use equations, presented here, contain the following exposure routes:
- incidental ingestion of soil or sediment
- dermal contact with soil or sediment
- inhalation of volatiles and particulates emitted from soil or sediment
- Total
A number of studies have shown that inadvertent ingestion of soil is common among children 6 years old and younger (Calabrese et al. 1989, Davis et al. 1990, Van Wijnen et al. 1990). Therefore, the dose method uses an age-adjusted soil ingestion factor that takes into account the difference in daily soil ingestion rates, body weights, and exposure duration for children from 1 to 6 years old and others from 7 to 26 years old. The equation is presented below. This health-protective approach is chosen to take into account the higher daily rates of soil ingestion in children as well as the longer duration of exposure that is anticipated for a long-term resident. For more on this method, see RAGS Part B (PDF) (68 pp, 721 K).
4.6.1.7 Supporting Equations
- Child
- Adult
- Age-adjusted
4.6.2 Recreator Surface Water
This receptor is exposed to chemicals that are present in surface water. Ingestion of water and dermal contact with water are appropriate pathways. Dermal contact with surface water is also considered for analytes determined to be within the effective predictive domain as described in Section 4.9.8. Inhalation is not considered due to mixing with outdoor air. There are no default RSLs for this scenario; only site-specific.
The recreator surface water land use is not provided in the Generic Tables but RSLs can be created by using the Calculator.
4.6.2.1 Noncarcinogenic - Child
The recreator surface water land use equation, presented here, contains the following exposure routes:
- incidental ingestion of water
- dermal
- Total
4.6.2.2 Noncarcinogenic - Adult
The recreator surface water land use equation, presented here, contains the following exposure routes:
- incidental ingestion of water
- dermal
- Total
4.6.2.3 Carcinogenic
The recreator surface water land use equation, presented here, contains the following exposure routes:
- incidental ingestion of water
- dermal
- Total
4.6.2.4 Mutagenic
The recreator surface water land use equation, presented here, contains the following exposure routes:
- incidental ingestion of water
- dermal
- Total
4.6.2.5 Vinyl Chloride - Carcinogenic
The recreator surface water land use equation, presented here, contains the following exposure routes:
- incidental ingestion of water
- dermal
- Total
4.6.2.6 Trichloroethylene - Carcinogenic and Mutagenic
The recreator surface water land use equation, presented here, contains the following exposure routes:
- incidental ingestion of water
- dermal
- Total
4.6.2.7 Supporting Equations
- Child
- Adult
- Age-adjusted
4.7 Ingestion of Fish
The fish RSL represents the concentration, in the fish, that can be consumed. Note: the consumption rate for fish is not age adjusted for this land use. Also, the SL calculated for fish is not for surface water or soil but is for fish tissue.
The ingestion of fish land use is not provided in the Generic Tables but RSLs can be created by using the Calculator.
4.7.1 Noncarcinogenic
The ingestion of fish equation, presented here, contains the following exposure route:
- consumption of fish.
4.7.2 Carcinogenic
The ingestion of fish equation, presented here, contains the following exposure route:
- consumption of fish
4.8 Soil to Groundwater
The soil to groundwater scenario was developed to identify concentrations in soil that have the potential to contaminate groundwater above risk based RSLs or MCLs. Migration of contaminants from soil to groundwater can be envisioned as a two-stage process: (1) release of contaminant from soil to soil leachate and (2) transport of the contaminant through the underlying soil and aquifer to a receptor well. The soil to groundwater scenario considers both of these fate and transport mechanisms. First, the acceptable groundwater concentration is multiplied by a dilution factor to obtain a target leachate concentration. For example, if the dilution factor is 10 and the MCL is 0.05 mg/L, the target soil leachate concentration would be 0.5 mg/L. The partition equation (presented in the Soil Screening Guidance documents) is then used to calculate the total soil concentration corresponding to this soil leachate concentration.
These equations are used to calculate screening levels in soil (SSLs) that are protective of groundwater. SSLs are either back-calculated from protective risk-based ground water concentrations or based on MCLs. The SSLs were designed for use during the early stages of a site evaluation when information about subsurface conditions may be limited. Because of this constraint, the equations used are based on conservative, simplifying assumptions about the release and transport of contaminants in the subsurface. Migration of contaminants from soil to groundwater can be envisioned as a two-stage process: (1) release of contaminant in soil leachate and (2) transport of the contaminant through the underlying soil and aquifer to a receptor well. The SSL methodology considers both of these fate and transport mechanisms.
The SSLs protective of groundwater, provided in the generic tables and the calculator, are all risk-based concentrations based on three phases (vapor, soil and water). No substitution for Csat is performed. If the risk-based concentration exceeds Csat, the resulting SSL concentration may be overly protective. This is because the dissolved, absorbed and vapor concentrations cease to rise linearly as soil concentration increases above the Csat level (pure product or nonaqueous phase liquid (NAPL) is present). The SSL model used in the RSL calculator is not a four phase model. If a NAPL is present at your site more sophisticated models may be necessary.
SSLs are provided for metals in the Generic Tables based on Kds from the Soil Screening Guidance Exhibit C-4 . According to Appendix C,
"Exhibit C-4 provides pH-specific soil-water partition coefficients (Kd) for metals. Site-specific soil pH measurements can be used to select appropriate Kd values for these metals. Where site-specific soil pH values are not available, values corresponding to a pH of 6.8 should be used."
If a metal is not listed in Exhibit C-4, Kds were taken from Baes, C. F. 1984 (PDF) (167 pp, 3.0 MB). Kds for organic compounds are calculated from Koc and the fraction of organic carbon in the soil (foc). Kds for metals are listed below.
This land use is for developing residential default soil screening levels for the protection of groundwater that are presented in the RSL Generic Tables.
Chemical | CAS or EPA ID |
Kd
|
Reference |
---|---|---|---|
Aluminum | 7429-90-5 |
1.50E+03
|
Baes, C.F. 1984 |
Antimony (metallic) | 7440-36-0 |
4.50E+01
|
SSG 9355.4-23 July 1996 |
Arsenic, Inorganic | 7440-38-2 |
2.90E+01
|
SSG 9355.4-23 July 1996 |
Barium | 7440-39-3 |
4.10E+01
|
SSG 9355.4-23 July 1996 |
Beryllium and compounds | 7440-41-7 |
7.90E+02
|
SSG 9355.4-23 July 1996 |
Boron And Borates Only | 7440-42-8 |
3.00E+00
|
Baes, C.F. 1984 |
Bromate | 15541-45-4 |
7.50E+00
|
Baes, C.F. 1984 |
Cadmium (Diet) | 7440-43-9 |
7.50E+01
|
SSG 9355.4-23 July 1996 |
Cadmium (Water) | 7440-43-9 |
7.50E+01
|
SSG 9355.4-23 July 1996 |
Chlorine | 7782-50-5 |
2.50E-01
|
Baes, C.F. 1984 |
Chromium (III) (Insoluble Salts) | 16065-83-1 |
1.80E+06
|
SSG 9355.4-23 July 1996 |
Chromium (VI) | 18540-29-9 |
1.90E+01
|
SSG 9355.4-23 July 1996 |
Chromium, Total | 7440-47-3 |
1.80E+06
|
SSG 9355.4-23 July 1996 |
Cobalt | 7440-48-4 |
4.50E+01
|
Baes, C.F. 1984 |
Copper | 7440-50-8 |
3.50E+01
|
Baes, C.F. 1984 |
Cyanide (CN-) | 57-12-5 |
9.90E+00
|
SSG 9355.4-23 July 1996 |
Fluoride | 16984-48-8 |
1.50E+02
|
Surrogate Value from Fluorine (Soluble Fluoride) |
Fluorine (Soluble Fluoride) | 7782-41-4 |
1.50E+02
|
Baes, C.F. 1984 |
Hydrogen Cyanide (HCN) | 74-90-8 |
9.90E+00
|
Surrogate value from Cyanide |
Iodine | 7553-56-2 | 6.0E+01 | Baes, C.F. 1984 |
Iron | 7439-89-6 |
2.50E+01
|
Baes, C.F. 1984 |
Lead and Compounds | 7439-92-1 |
9.00E+02
|
Baes, C.F. 1984 |
Lithium | 7439-93-2 |
3.00E+02
|
Baes, C.F. 1984 |
Magnesium | 7439-95-4 |
4.50E+00
|
Baes, C.F. 1984 |
Manganese (Diet) | 7439-96-5 |
6.50E+01
|
Baes, C.F. 1984 |
Manganese (Water) | 7439-96-5 |
6.50E+01
|
Baes, C.F. 1984 |
Mercury (elemental) | 7439-97-6 |
5.20E+01
|
SSG 9355.4-23 July 1996 |
Methyl Mercury | 22967-92-6 |
7.0E+02
|
EPA Mercury Study Report to Congress |
Molybdenum | 7439-98-7 |
2.00E+01
|
Baes, C.F. 1984 |
Nickel Refinery Dust | E715532 | 1.5E+02 | Baes, C.F. 1984 |
Nickel Soluble Salts | 7440-02-0 |
6.50E+01
|
SSG 9355.4-23 July 1996 |
Phosphorus, White | 7723-14-0 |
3.50E+00
|
Baes, C.F. 1984 |
Selenium | 7782-49-2 |
5.00E+00
|
SSG 9355.4-23 July 1996 |
Silver | 7440-22-4 |
8.30E+00
|
SSG 9355.4-23 July 1996 |
Sodium | 7440-23-5 |
1.00E+02
|
Baes, C.F. 1984 |
Sodium Fluoride | 7681-49-4 |
1.50E+02
|
Surrogate Value from Fluorine (Soluble Fluoride) |
Strontium, Stable | 7440-24-6 |
3.50E+01
|
Baes, C.F. 1984 |
Thallium (Soluble Salts) | 7440-28-0 |
7.10E+01
|
SSG 9355.4-23 July 1996 |
Tin | 7440-31-5 |
2.50E+02
|
Baes, C.F. 1984 |
Tungsten | 7440-33-7 |
1.5E+02
|
Baes, C.F. 1984 |
Uranium | 7440-62-2 |
4.50E+02
|
Baes, C.F. 1984 |
Vanadium and Compounds | 7440-62-2 |
1.00E+03
|
SSG 9355.4-23 July 1996 |
Vanadium, Metallic | 7440-62-2 |
1.00E+03
|
SSG 9355.4-23 July 1996 |
Zinc (Metallic) | 7440-66-6 |
6.20E+01
|
SSG 9355.4-23 July 1996 |
Zirconium | 7440-67-7 |
3.00E+03
|
Baes, C.F. 1984 |
Because Kds vary greatly by soil type, it is highly recommended that site-specific Kds be determined and used to develop SSLs.
The more protective of the carcinogenic and noncarcinogenic SLs is selected to calculate the SSL.
4.8.1 Noncarcinogenic Tapwater Equations for SSLs
The tapwater equations, presented in Section 4.1.2.1, are used to calculate the noncarcinogenic SSLs for volatiles and nonvolatiles. If the contaminant is a volatile, ingestion, dermal and inhalation exposure routes are considered. If the contaminant is not a volatile, only ingestion and dermal are considered.
4.8.2 Carcinogenic Tapwater Equations for SSLs
The tapwater equations, presented in Section 4.1.2.3, are used to calculate the carcinogenic SSLs for volatiles and nonvolatiles. Sections 4.1.2.4 and 4.1.2.5 present the mutagenic and vinyl chloride equations, respectively. If the contaminant is a volatile, ingestion, dermal and inhalation exposure routes are considered. If the contaminant is not a volatile, only ingestion and dermal are considered.
4.8.3 Method 1 for SSL Determination
Method 1 employs a partitioning equation for migration to groundwater and defaults are provided. This method is used to generate the download default tables. If H' is not available, SSL can still be calculated. H' changes when groundwater temperature changes. Please see section 4.9.9 of this user guide for H' determination at temperature other than 25°C.
- method 1.
The fraction of organic carbon (foc) selected for this equation is 0.002. This is the default for subsurface soil identified in U.S. EPA 1996b, Sections 2.5.2 and 2.5.7. According to this source, soil organic carbon decreases rapidly with depth. Note that the default foc in section “4.9.4 Infinite Source Chronic Volatilization Factor (VFulim)” is 0.006, which is the default for surface soil from the same study.
4.8.4 Method 2 for SSL Determination
Method 2 employs a mass-limit equation for migration to groundwater and site-specific information is required. This method can be used in the calculator portion of this website.
- method 2.
4.8.5 Determination of the Dilution Factor
The SSL values in the download tables are based on a dilution factor of 1. If one wishes to use the calculator to calculate screening levels using the SSL guidance for a source up to 0.5 acres, then a dilution factor of 20 can be used. If all of the parameters needed to calculate a site-specific dilution factor are known, they may be entered.
- Dilution Attenuation Factor.
4.8.6 Conservative and Simplifying Assumptions for the Soil to Groundwater Scenario
The SSL scenario was designed for use during the early stages of a site evaluation when information about subsurface conditions may be limited. Because of this constraint, methods 1 and 2 are based on conservative, simplifying assumptions about the release and transport of contaminants in the subsurface. These assumptions include:
- The source is infinite (i.e., steady-state concentrations will be maintained in ground water over the exposure period of interest).
- Contaminants are uniformly distributed throughout the zone of contamination.
- Soil contamination extends from the surface to the water table (i.e., adsorption sites are filled in the unsaturated zone beneath the area of contamination).
- There is no contaminant loss due to biological degradation or volatilization in the unsaturated zone.
- Equilibrium soil/water partitioning is instantaneous and linear in the contaminated soil.
- The receptor well is at the edge of the source (i.e., there is no dilution from recharge downgradient of the site) and is screened within the plume.
- The aquifer is unconsolidated and unconfined (surficial).
- Aquifer properties are homogeneous and isotropic.
- Chelating or complexing agents not present.
- No facilitated transport (e.g., colloidal transport) of inorganic contaminants in aquifer.
RSLs developed using this methodology can be viewed as evolving risk-based levels that can be refined as more site information becomes available. The early use of the methodology at a site will help focus further subsurface investigations on areas of true concern with respect to ground water quality and will provide information on soil characteristics, aquifer characteristics, and contaminant properties that can be built upon as a site evaluation progresses.
4.9 Supporting Equations and Parameter Discussion
There are two parts of the above land use equations that require further explanation. They are the inhalation variables: the particulate emission factor (PEF) and the volatilization factor (VF).
4.9.1 Wind-driven Particulate Emission Factor (PEF)
Inhalation of contaminants adsorbed to respirable particles (PM10) was assessed using a default PEF equal to 1.36 x 109 m3/kg. This equation relates the contaminant concentration in soil with the concentration of respirable particles in the air due to fugitive dust emissions from contaminated soils. The generic PEF was derived using default values that correspond to a receptor point concentration of approximately 0.76 µg/m3. The relationship is derived by Cowherd (1985) for a rapid assessment procedure applicable to a typical hazardous waste site, where the surface contamination provides a relatively continuous and constant potential for emission over an extended period of time (e.g., years). This represents an annual average emission rate based on wind erosion that should be compared with chronic health criteria; it is not appropriate for evaluating the potential for more acute exposures. Definitions of the input variables are in Table 1.
With the exception of specific heavy metals, the PEF does not appear to significantly affect most soil screening levels. The equation forms the basis for deriving a generic PEF for the inhalation pathway. For more details regarding specific parameters used in the PEF model, refer to Appendix D of the Supplemental Soil Screening Guidance. The use of alternate values on a specific site should be justified and presented in an Administrative Record if considered in CERCLA remedy selection.
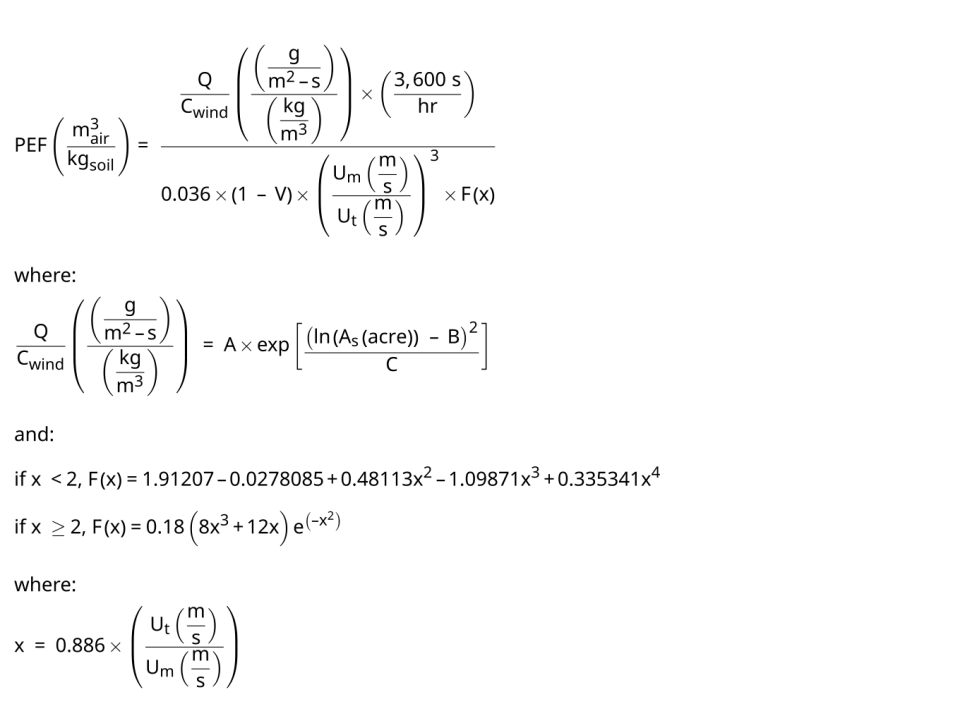
Note: the generic PEF evaluates wind-borne emissions and does not consider dust emissions from traffic or other forms of mechanical disturbance that could lead to greater emissions than assumed here.
4.9.2 Vehicle traffic-driven Particulate Emission Factor (PEFsc)
The equation to calculate the subchronic particulate emission factor (PEFsc) is significantly different from the residential and non-residential PEF equations. The PEFsc focuses exclusively on emissions from truck traffic on unpaved roads, which typically contribute the majority of dust emissions during construction. This equation requires estimates of parameters such as the number of days with at least 0.01 inches of rainfall, the mean vehicle weight, and the sum of fleet vehicle distance traveled during construction.
The number of days with at least 0.01 inches of rainfall can be estimated using Exhibit 5-2 in the supplemental soil screening guidance (PDF) (187 pp, 2.2 MB). Mean vehicle weight (W) can be estimated by assuming the numbers and weights of different types of vehicles. For example, assuming that the daily unpaved road traffic consists of 20 two-ton cars and 10 twenty-ton trucks, the mean vehicle weight would be:
W = [(20 cars x 2 tons/car) + (10 trucks x 20 tons/truck)]/30 vehicles = 8 tons
The sum of the fleet vehicle kilometers traveled during construction (∑ VKT) can be estimated based on the size of the area of surface soil contamination, assuming the configuration of the unpaved road, and the amount of vehicle traffic on the road. For example, if the area of surface soil contamination is 0.5 acres (or 2,024 m2), and one assumes that this area is configured as a square with the unpaved road segment dividing the square evenly, the road length would be equal to the square root of 2,024 m2, 45 m (or 0.045 km). Assuming that each vehicle travels the length of the road once per day, 5 days per week for a total of 6 months, the total fleet vehicle kilometers traveled would be:
∑ VKT = 30 vehicles x 0.045 km/day x (52 weeks/year ÷ 2) x 5 days/wk = 175.5 km
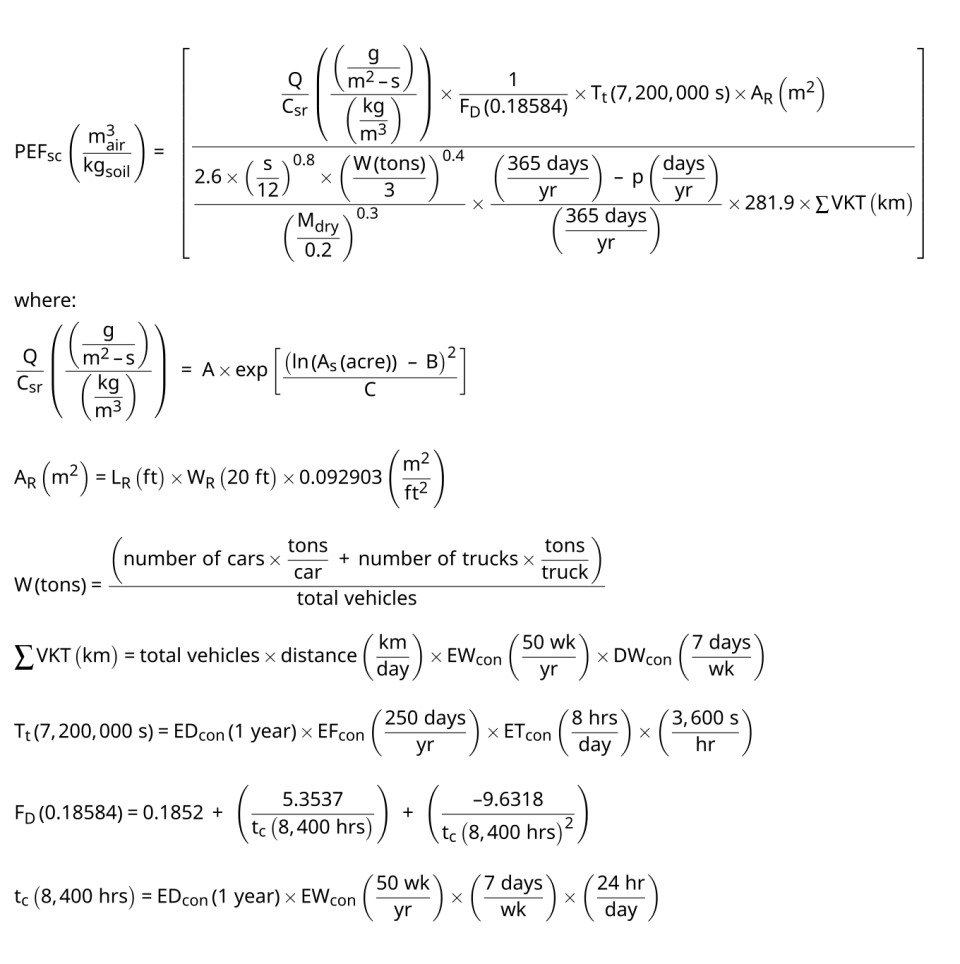
4.9.3 Other than vehicle traffic-driven Particulate Emission Factor (PEF'sc)
Other than emissions from unpaved road traffic, the construction worker may also be exposed to particulate matter emissions from wind erosion, excavation soil dumping, dozing, grading, and tilling or similar operations PEF'sc. These operations may occur separately or concurrently and the duration of each operation may be different. For these reasons, the total unit mass emitted from each operation is calculated separately and the sum is normalized over the entire area of contamination and over the entire time during which construction activities take place. Equation E-26 in the supplemental soil screening guidance (PDF) (187 pp, 2.2 MB) was used.
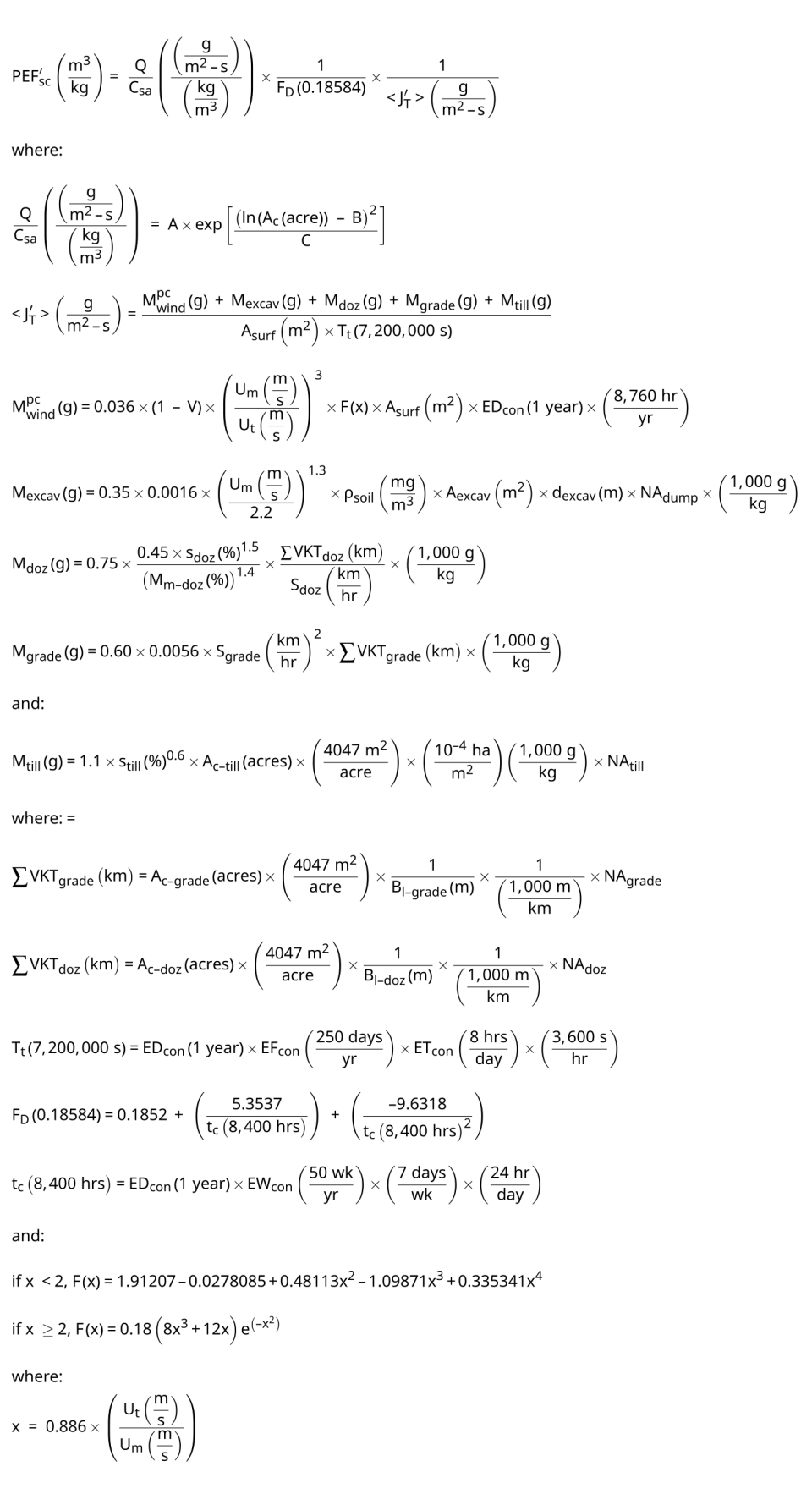
4.9.4 Infinite Source Chronic Volatilization Factor (VFulim)
The soil-to-air VF is used to define the relationship between the concentration of the contaminant in soil and the flux of the volatilized contaminant to air. VF is calculated from the equation below using chemical-specific properties and either site-measured or default values for soil moisture, dry bulk density, and fraction of organic carbon in soil. If H' is not available, VFulim can still be calculated. The Soil Screening Guidance: User's Guide (PDF) (89 pp, 863 K) describes how to develop site measured values for these parameters.
VF is only calculated for volatile compounds. Volatiles, for the purpose of the RSLs, are chemicals with a Henry's Law constant greater than 1 x 10-5 atm-m3/mole or a vapor pressure greater than 1 mm Hg. This definition of volatility is taken from https://www.epa.gov/sites/default/files/2015-09/documents/oswer-vapor-intrusion-technical-guide-final.pdf. The volatile status of a chemical is important for some exposure routes. According to RAGS Part E, dermal absorption to soil is not assessed for volatiles. For the purposes of this guidance, dermal exposure to soil is only quantified if RAGS Part E provides a dermal absorption value in Exhibit 3-4 or the website, regardless of volatility status. The rationale for this is that in the considered soil exposure scenarios, volatile organic compounds would tend to be volatilized from the soil on skin and should be accounted for via inhalation routes in the combined exposure pathway analysis. Further, a chemical must be volatile in order to be included in the calculation of tapwater inhalation. H' changes when groundwater temperature changes. Please see section 4.9.9 of this user guide for H' determination at temperature other than 25°C.
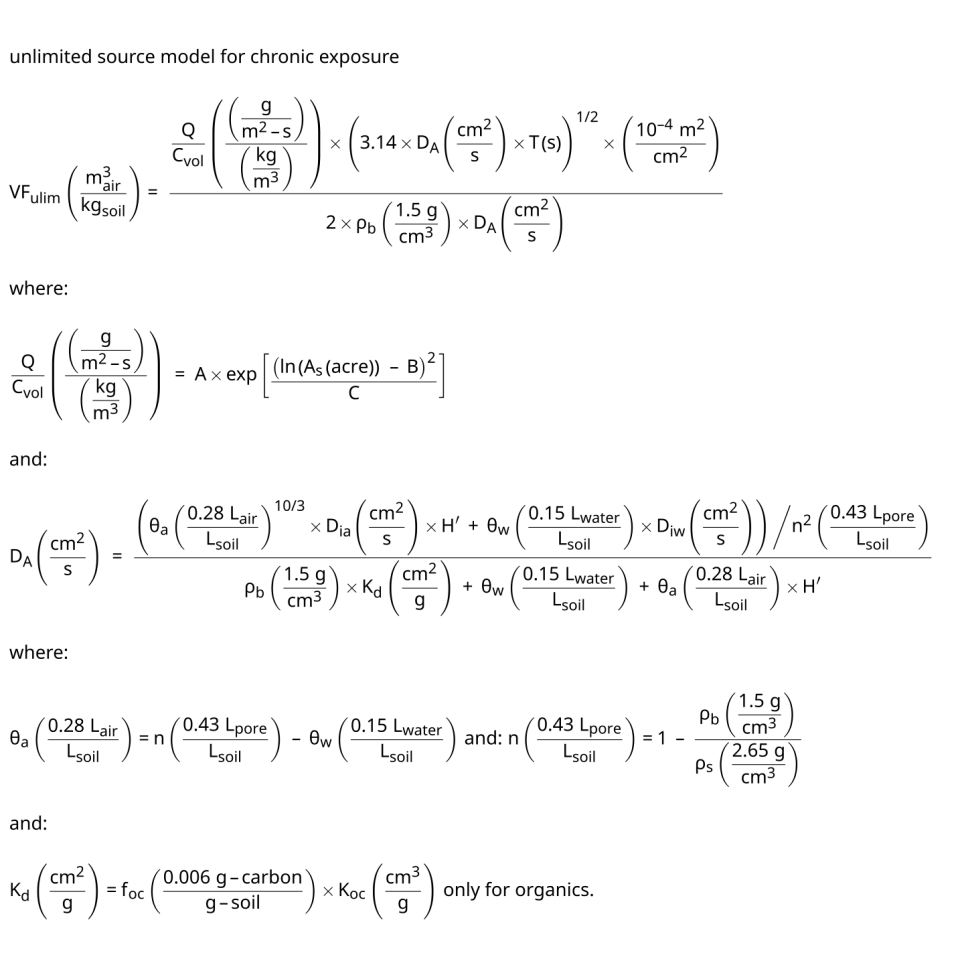
The fraction of organic carbon (foc) selected for this equation is 0.006. This is the default for surface soil identified in U.S. EPA 1996b, Sections 2.4.2 and 2.5.7, and represents the mean value for the top 0.3m of Class B soils. According to this source, soil organic carbon decreases rapidly with depth. Note that the default foc in section “4.8.3 Method 1 for SSL Determination” is 0.002, which is the default for subsurface soil from the same study.
Diffusivity in Water (cm2/s)
Diffusivity in water can be calculated from the chemical's molecular weight and density, using the following correlation equation based on WATER9 (U.S. EPA, 2001 (PDF) (38 pp, 185 K)):
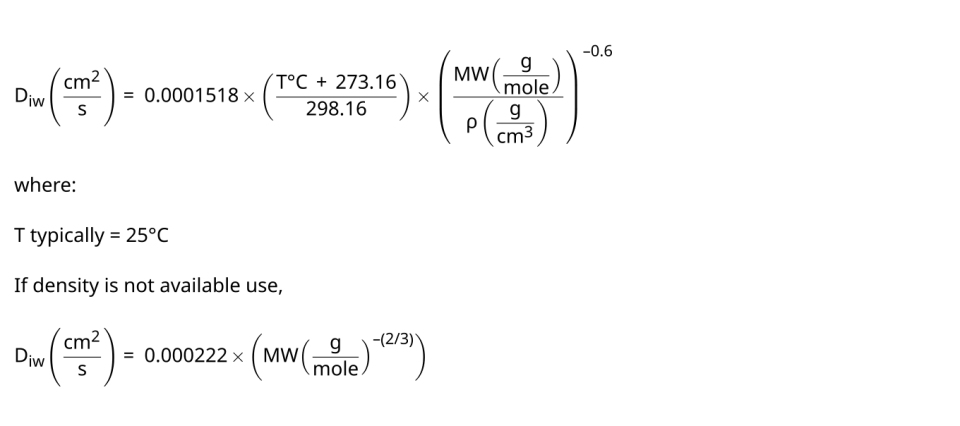
If density is not available, diffusivity in water can be calculated using the correlation equation based on U.S. EPA (1987). The value for diffusivity in water must be greater than zero. No maximum limit is enforced.
Diffusivity in Air (cm2/s).
Diffusivity in air can be calculated from the chemical's molecular weight and density, using the following correlation equation based on WATER9 (U.S. EPA, 2001 (PDF) (38 pp, 185 K)). If density is not available, an alternate equation is provided.:
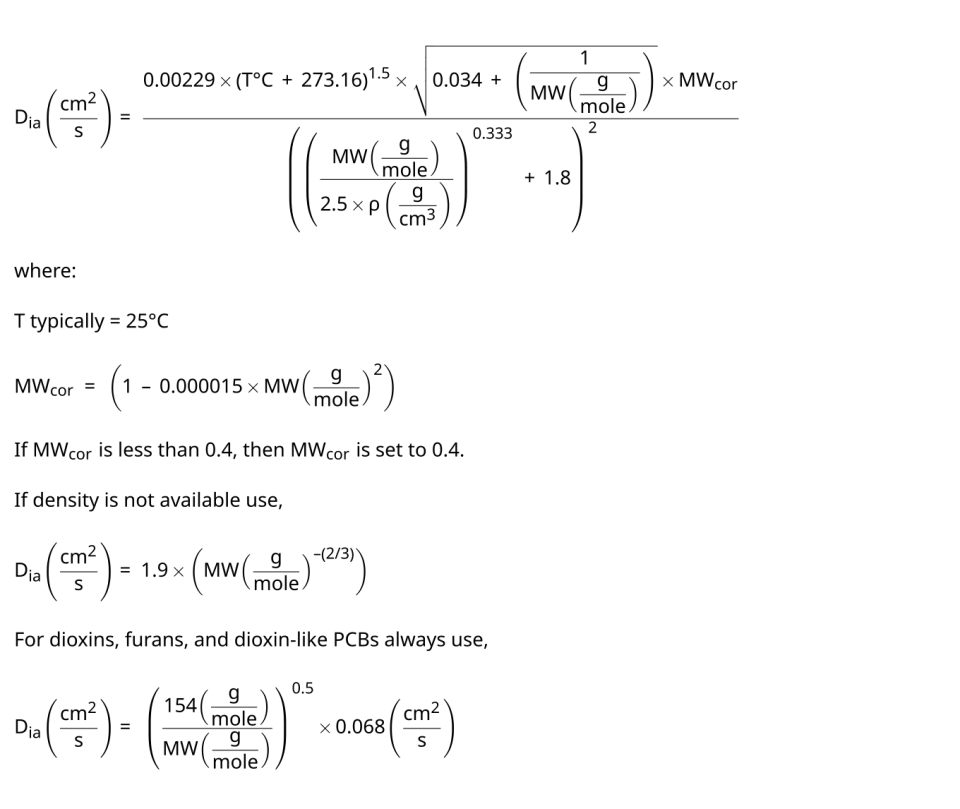
For dioxins, furans, and dioxin-like PCBs, diffusivity in air should always be calculated from the molecular weight using the Graham's Law correlation equation based on December 2003 NAS Review Draft Part I: Volume 3 (pg 4-38 )(PDF) (148 pp, 1.9 MB). In this equation, the unknown diffusivity is solved by correlation to the known diphenyl diffusivity of 0.068 cm2/s and MW of 154 g/mol.
4.9.5 Mass-limit Chronic Volatilization Factor (VFmlim)
This Equation presents a model for calculating mass-limit SSLs for the outdoor inhalation of volatiles. This model can be used only if the depth and area of contamination are known or can be estimated with confidence. This equation is presented in the Soil Screening Guidance: User's Guide (PDF) (89 pp, 863 KF) and the Supplemental Soil Screening Guidance (PDF) (187 pp, 2.2 MB).
Use of infinite source models to estimate volatilization can violate mass balance considerations, especially for small sources. To address this concern, the Soil Screening Guidance includes a model for calculating a mass-limit SSL that provides a lower limit to the SSL when the area and depth (i.e., volume) of the source are known or can be estimated reliably.
A mass-limit SSL represents the level of contaminant in the subsurface that is still protective when the entire volume of contamination volatilizes over the 26-year exposure duration and the level of contaminant at the receptor does not exceed the health-based limit.
To use mass-limit SSLs, determine the area and depth of the source, calculate both standard and mass-limit SSLs, compare them for each chemical of concern and select the higher of the two values.
Note that the equation requires a site-specific determination of the average depth of contamination in the source. Step 3, in the SSG, provides guidance for conducting subsurface sampling to determine source depth. Where the actual average depth of contamination is uncertain, a conservative estimate should be used (e.g., the maximum possible depth in the unsaturated zone). At many sites, the average water table depth may be used unless there is reason to believe that contamination extends below the water table. In this case SSLs do not apply and further investigation of the source in question is needed.
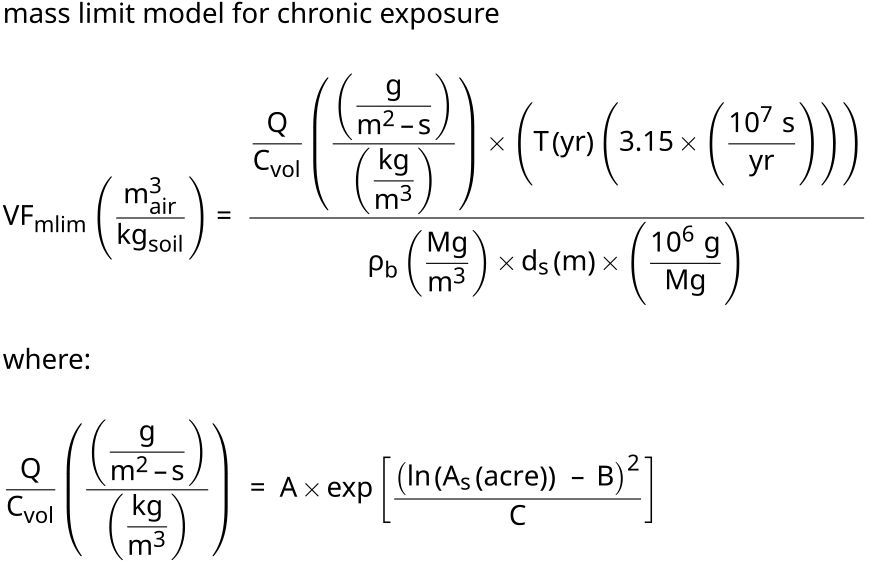
4.9.6 Unlimited Source Subchronic Volatilization Factor for Construction Worker (VFulim-sc)
Equation 5-14 of the supplemental soil screening guidance (PDF) (187 pp, 2.2 MB) is appropriate for calculating the soil-to-air volatilization factor (VFulim-sc) that relates the concentration of a contaminant in soil to the concentration in air resulting from volatilization. The equation for the subchronic dispersion factor for volatiles, Q/Csa, is presented in Equation 5-15 of the supplemental soil screening guidance (PDF) (187 pp, 2.2 MB). Q/Csa was derived using EPA's SCREEN3 dispersion model for a hypothetical site under a wide range of meteorological conditions. Unlike the Q/C values for the other scenarios, the Q/Csa for the construction scenario's simple site-specific approach can be modified only to reflect different site sizes between 0.5 and 500 acres; it cannot be modified for climatic zone. Site managers conducting a detailed site-specific analysis for the construction scenario can develop a site-specific Q/C value by running the SCREEN3 model. If H' is not available, VFulim-sc can still be calculated. Further details on the derivation of Q/Csa can be found in Appendix E (PDF) (42 pp, 779 K) of the supplemental soil screening guidance (PDF) (187 pp, 2.2 MB). If H' is not available, DA can still be calculated. H' changes when groundwater temperature changes. Please see section 4.9.9 of this user guide for H' determination at temperature other than 25°C.
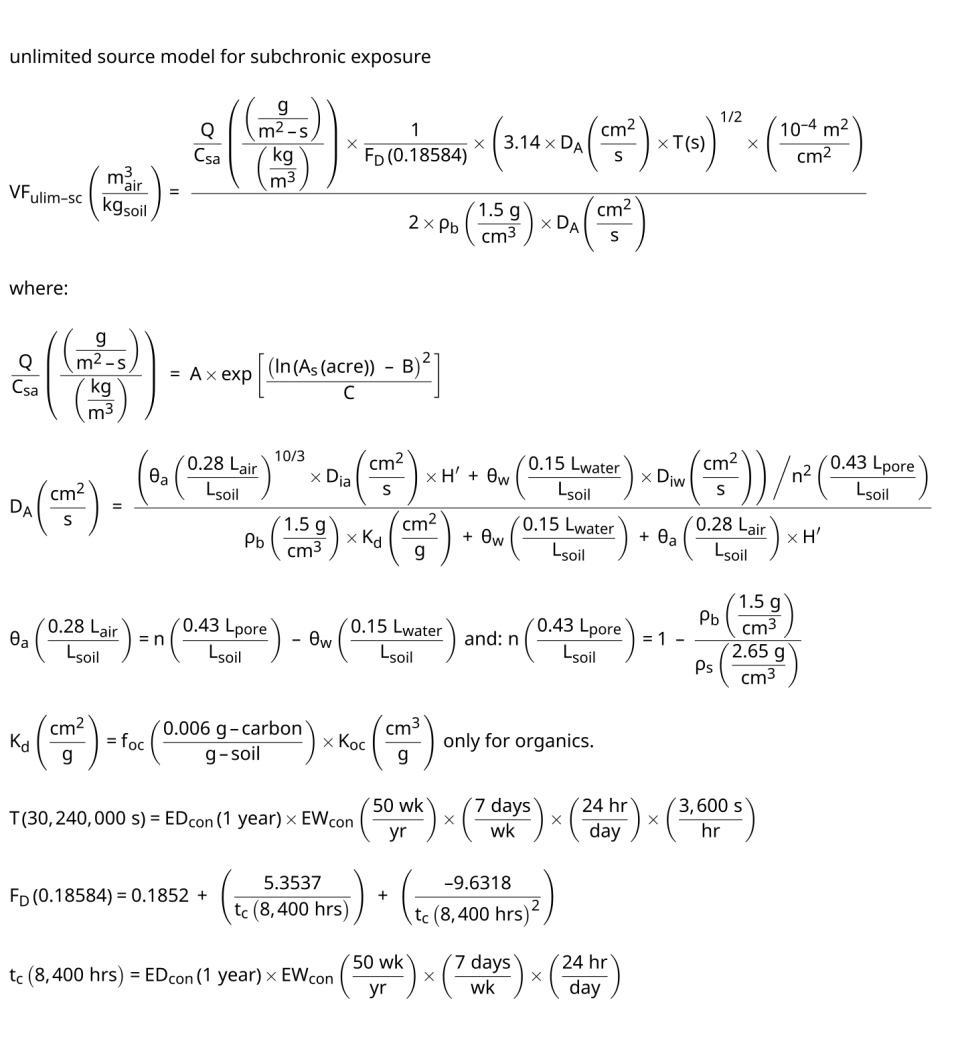
4.9.7 Mass-limit Subchronic Volatilization Factor for Construction Worker (VFmlim-sc)
Because the equations developed to calculate SSLs for the inhalation of volatiles outdoors assume an infinite source, they can violate mass-balance considerations, especially for small sources. To address this concern, a mass-limit SSL equation for this pathway may be used (Equation 5-17 of the supplemental soils screening guidance (PDF) (187 pp, 2.2 MB)). This equation can be used only when the volume (i.e., area and depth) of the contaminated soil source is known or can be estimated with confidence. As discussed above, the simple site-specific approach for calculating construction scenario SSLs uses the same emission model for volatiles as that used in the residential and non-residential scenarios. However, the conservative nature of this model (i.e., it assumes all contamination is at the surface) makes it sufficiently protective of construction worker exposures to volatiles.
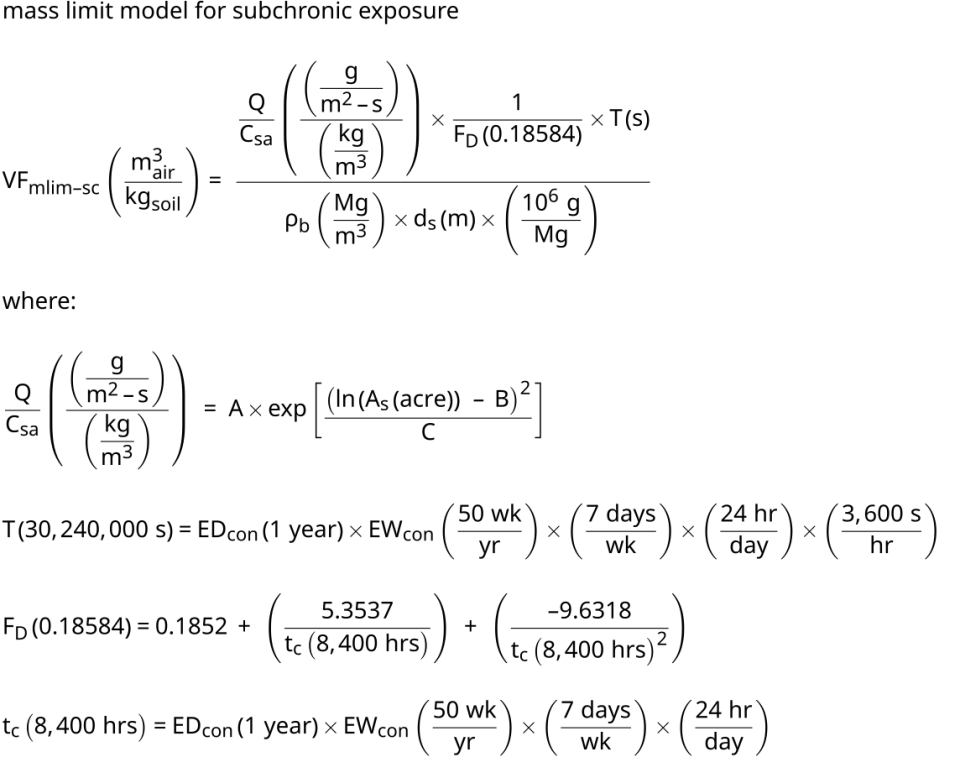
4.9.8 Dermal Contact with Water Supporting Equations
RAGS Part E stresses the determination of whether a chemical is within the effective predictive domain (EPD) for the determination of dermal permeability constant (Kp) applicability. The RSL output provides determination of whether or not a chemical is within the EPD. The RSL output also displays our determination of the fraction of the chemical that is ultimately absorbed (FA). FA depends strongly on the chemical's lipophilic characteristic and molecular weight as expressed in the B parameter and the lag time. RAGS Part E has determined that dermal exposures contributing less than 10% of oral exposures are insignificant. However, the RSL presents dermal screening levels regardless of contribution percent.
-
Effective Predictive Domain (EPD) is an area on a X/Y plot that symbolizes 95% statistical confidence levels of a regression equation to accurately estimate a dermal permeability constant (Kp). Only if a chemical is within the EPD, will the dermal route be calculated. The EPD is determined by investigating the predictive power of a regression equation using MW and log Kow values for a compound. If the intersection of the values falls within the designated plotted area, the chemical is determined to be in the EPD, and the dermal route is calculated. The boundaries of MW and log Kow for the regression equation are presented below. The EPD is depicted in RAGS Part E in Appendix A; Exhibit A-1.
-
Dermal permeability constant (Kpcm/hr) values are either from EPI Suite or RAGS Part E in the chemical parameter hierarchy. Values from both sources are estimated. Each source has a different estimation model. The RAGS Part E model requires a log Kow and a MW, and if both log Kow and MW are available, a Kp is calculated. The RAGS Part E model is taken from the spreadsheet that accompanies RAGS Part E documentation and is reproduced below.
- Fraction absorbed water (FA) is described in RAGS Part E in Appendix A. The FA term should be applied to account for the loss of chemical due to the desquamation of the outer skin layer and a corresponding reduction in the absorbed dermal dose. To determine FA values for the RSLs, the following regression analysis is performed. This analysis builds on the RAGS Part E data.
- The dimensionless value (B) is the ratio of the permeability coefficient of a compound through the stratum corneum relative to its permeability coefficient across the viable epidermis (ve).
-
t* = Time to reach steady-state (hours) = 2.4 τevent
-
τevent = Lag time per event (hours/event)
4.9.9 Henry's Law constant and Vapor Pressure Determination at Temperature Other Than 25°C
In site-specific mode for soil and soil to groundwater land uses, users are given the option to the change groundwater-soil system temperature from the default of 25°C to a site-specific value. Since the unitless Henry's Law Constant (H') is derived based on the partial pressure of a gas in equilibrium with a liquid and the equilibrium changes when temperature changes, Henry's Law is changed to reflect the equilibrium at the given temperature. The equation below illustrates how H' is derived when groundwater-soil system temperature is changed. An EPA Fact Sheet describing the process can be found at Henrys Law Constant Fact Sheet.
Use the map below to find the average groundwater temperature in your region.
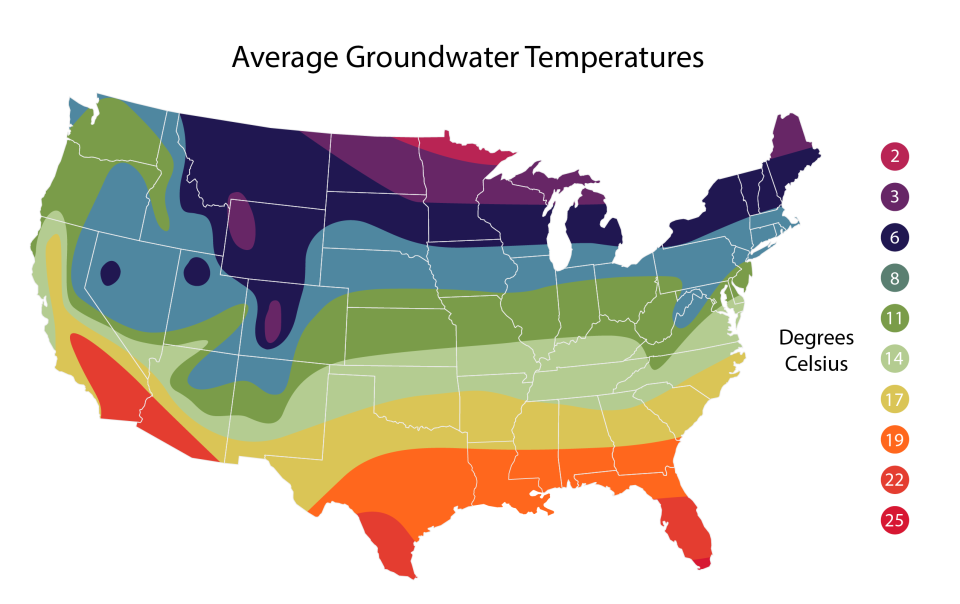
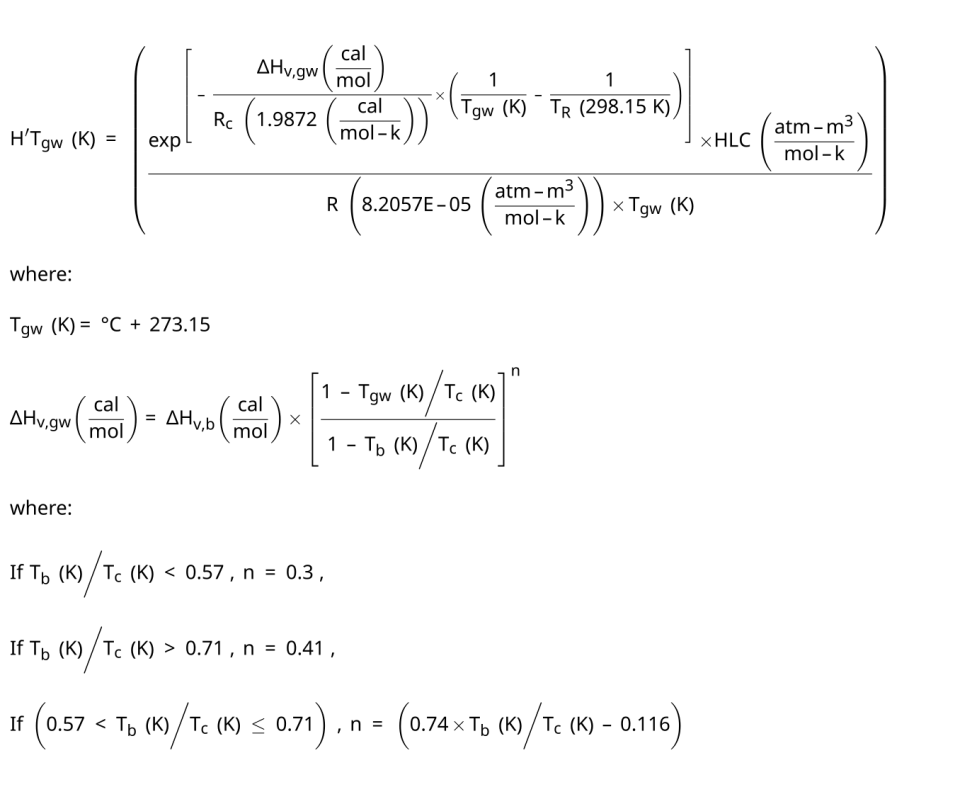
The equation below illustrates how vapor pressure is derived when groundwater-soil system temperature is changed.
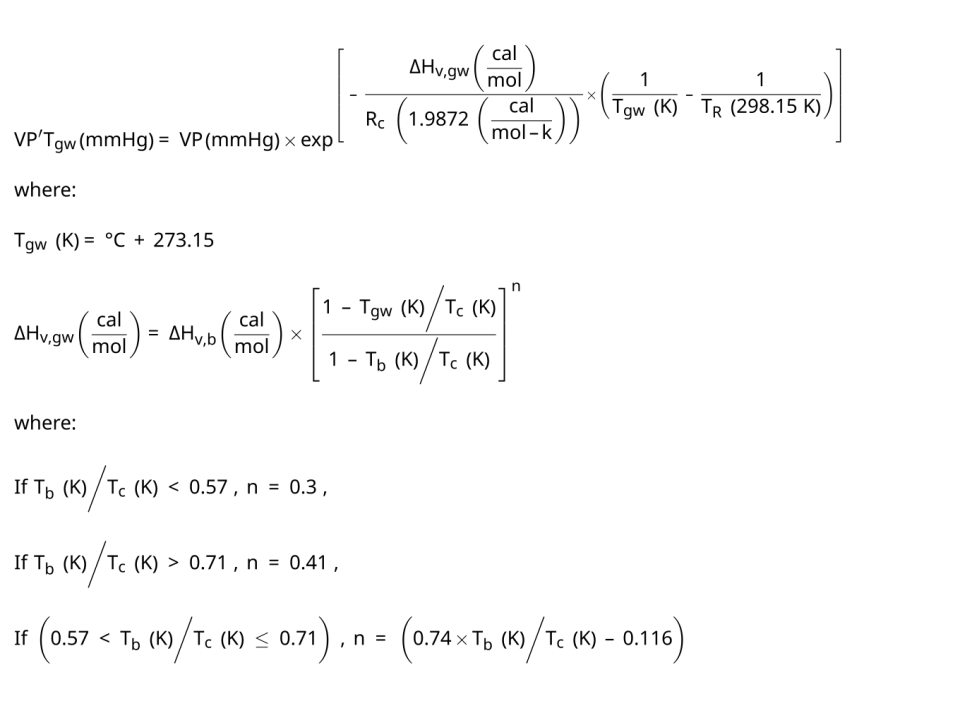
When changing the groundwater-soil system temperature, the criteria that determines whether a chemical is volatile or not may also change. The Henry’s Law constants and vapor pressures that are used in RSL calculations are based on standard laboratory conditions of 25°C. When the groundwater-soil system temperature is changed, the Henry’s Law constants and vapor pressures will change accordingly. A volatile chemical at 25°C may not be volatile at a lower temperature. Conversely, a nonvolatile may become volatile at temperatures above 25°C. When the groundwater-soil system temperature is changed the RSL calculator will provide the user with recalculated Henry's Law constants and vapor pressures. These values can be compared to the volatility status requirements presented in section 4.9.4. The RSL calculator does not automatically change the volatility status of a chemical to reflect the user's change of the groundwater-soil system temperature. In site-specific user-provided mode of the calculator, the user may change the volatility status of a chemical if deemed appropriate by the user’s regional risk assessor. Changing the volatility status can impact the inclusion of dermal exposure to soil, inhalation of volatiles during household use of water, and the soil to groundwater Method 1 calculations. See section 4.9.4 and 4.8.3 for more information.
5. Special Considerations
Most of the SLs are readily derived by referring to the above equations. However, there are some cases for which the standard equations do not apply and/or external adjustments to the SLs are recommended. These special case chemicals are discussed below.
5.1 Cadmium
"Cadmium (Diet)" is for food and soil use; "Cadmium (Water)" is for water use only. The diet RfD incorporates a 2.5% gastrointestinal absorption (GIABS) adjustment; the water RfD incorporates a 5% GIABS. These GIABS values are used in the RSL calculations to convert oral RfDs to Dermal RfDs, as directed by EPA Risk Assessment Guidance for Superfund (RAGS) Part E, Supplemental Guidance for Dermal Risk Assessment July 2004. Document (PDF) (186 pp, 4.2 MB) and website.
The RSLs use the chronic MRL from ATSDR as the Cadmium RfD instead of the IRIS value in response to an EPA memo "Recommendations on the Use of Chronic or Subchronic Noncancer Values for Superfund Human Health Risk Assessments" released in May 2021; this memo recommends using lower tier toxicity values that are more recent, credible, and relevant than the values that would be automatically selected from the RSL toxicity hierarchy. In this memo, it was recommended that the ATSDR chronic RfD of 1E-04 mg/kg-day from 2012 be used over the IRIS values (5E-04 and 1E-03 mg/kg-day for water and diet, respectively) from 1989. The RSLs have adopted this recommendation and retain the GIABS values from RAGS Part E.
5.2 Lead
EPA has no consensus RfD or SFO for inorganic lead, so it is not possible to calculate SLs using the same methodology used for other chemicals. EPA considers lead to be a special case because of the difficulty in identifying a level of exposure, or dose, below which adverse effects are unlikely, which is needed to develop a RfD.
EPA evaluates lead exposure in residential scenarios by using models such as the Integrated Exposure-Uptake Biokinetic Model (IEUBK) to estimate a blood-lead concentration. Since 1994, the EPA Office of Land and Emergency Management (formerly known as OSWER) has released several directives on risk assessment and screening levels for residential soil lead. The most recent guidance, Updated Soil Lead Guidance for CERCLA Sites and RCRA Corrective Action Facilities (2024), recommends a residential soil lead screening level of 200 mg/kg (ppm) where children live and play. However, the guidance indicates that for remedial responses, the residential soil screening level should be lowered to 100 mg/kg where there is aggregate exposure and increased risk to children living in communities with multiple sources of lead contamination, such as lead service lines, lead-based paint, or non-attainment areas where the lead concentrations in air exceed the National Ambient Air Quality Standards. A screening level for soil lead at commercial/industrial (non-residential) sites is 800 mg/kg. More information can be found here. EPA recommends using the Office of Water Action Level of 10 µg/L in water and the National Ambient Air Quality Standard of 0.15 µg/m3 in air for Screening levels.
EPA uses the Adult Lead Methodology (ALM) model to estimate SLs for an industrial setting. This SL is intended to protect a fetus that may be carried by a pregnant worker. A primary assumption of this methodology is that a screening level that is protective of a fetus will also afford protection for male or female adult workers. The ALM model equations were developed to calculate screening levels such that the fetus of a pregnant worker would not likely have an unsafe concentration of lead in blood.
The default values for absolute bioavailability (ABA) in the IEUBK model for lead in children are 0.3 for soil and dust, and 0.5 for food and water. This corresponds to an RBA for soil of 0.6 (ABA_soil / ABA_water = 0.6). It’s important to note that the ABA values in the IEUBK model are central estimates, and the oral RBA at any given site may be higher or lower than the default oral RBA for lead. For this reason, and because it provides a more comprehensive characterization of exposure at a site, the TRW recommends using EPA SW-846 Method 1340 to estimate the site-specific RBA in soil. Guidance related to these topics can be found in the Soil Bioavailability at Superfund Site Guidance.
For more information on EPA's lead models and other lead-related topics, please go to Addressing Lead at Superfund Sites.
5.3 Manganese
The IRIS RfD (0.14 mg/kg-day) includes manganese from all sources, including diet. The author of the IRIS assessment for manganese recommended that the dietary contribution from the normal U.S. diet (an upper limit of 5 mg/day) be subtracted when evaluating non-food (e.g., drinking water or soil) exposures to manganese, leading to a RfD of 0.071 mg/kg-day for non-food items. The explanatory text in IRIS further recommends using a modifying factor of 3 when calculating risks associated with non-food sources due to a number of uncertainties that are discussed in the IRIS file for manganese, leading to a RfD of 0.024 mg/kg-day. This modified RfD has been used in the derivation of some manganese screening levels for soil and water. For more information regarding the Manganese RfD, users are advised to contact the author of the IRIS assessment on Manganese.
5.4 Vanadium Compounds
The oral RfD toxicity value for Vanadium, used in this website, is derived from the IRIS oral RfD for Vanadium Pentoxide by factoring out the molecular weight (MW) of the oxide ion. Vanadium Pentoxide (V205) has a molecular weight of 181.88. The two atoms of Vanadium contribute 56% of the MW. Vanadium Pentoxide's oral RfD of 9E-03 mg/kg-day multiplied by 56% gives a Vanadium oral RfD of 5.04E-03 mg/kg-day.
5.5 Uranium
The "Uranium Soluble Salts" RSL uses the ATSDR intermediate MRL of 2E-04 mg/kg-day instead of the IRIS oral RfD of 3E-03 mg/kg-day. This is a deviation from the typical RSL toxicity hierarchy. This deviation was justified by the 2003 hierarchy memo (PDF) (4 pp, 25 K) that acknowledges and "recognizes that EPA should use the best science available on which to base risk assessments." In December 2016, the EPA Office of Superfund Remediation and Technology Innovation (OSRTI) announced its determination that the ATSDR intermediate MRL generally reflects a better scientific basis for assessing the chronic health risks of soluble uranium than the RfD currently available in IRIS." The rationale for this determination is summarized in an accompanying memorandum (PDF) (11 pp, 2.5 MB), which recommends use of the ATSDR intermediate MRL for assessing chronic and subchronic human exposures at Superfund sites nationwide.
5.6 Chromium (VI)
It is recommended that valence-specific data for chromium be collected when chromium is likely to be an important contaminant at a site and when hexavalent chromium (Cr (VI)) may be present. The 2024 IRIS toxicity profile identifies Cr(VI) as carcinogenic via a mutagenic mode of action. Therefore, the RSLs will be applying Age-Dependent Adjustment Factors (ADAFs) to the cancer toxicity values provided in the assessment. The 2024 IRIS assessment is based solely on Cr(VI); therefore, the adjustment to modify total chromium exposure to Cr(VI) using a 6:1 ratio of Cr(III):Cr(VI) is no longer necessary. The Maximum Contaminant Level (MCL) of 100 µg/L for "Chromium (total)" from the EPA's MCL listing is applied to the "Chromium, Total" analyte on this website.
5.7 Aminodinitrotoluenes
The IRIS oral RfD of 2E-03 mg/kg-day for 2,4-Dinitrotoluene is no longer used as a surrogate for 2-Amino-4,6-Dinitrotoluene and 4-Amino-2,6-Dinitrotoluene. Appendix screening values from a new PPRTV assessment are being used instead.
5.8 PCBs and Aroclors
In the RSL Tables, the chemical group, "Polychlorinated Biphenyls (PCBs)," has three distinct types of PCBs listed: Aroclors, individual PCB congeners, and risk/persistence-based PCBs. The PCB congeners are evaluated as dioxin-like, using dioxin TEFs, as discussed in section 2.3. The risk/persistence-based PCB toxicity values come from the IRIS summary. In that summary, high, low, and lowest risk and persistence human oral slope factor tiers are given. Below is the RSL interpretation of this information and is represented in the RSL tables and calculator.
TIERS OF HUMAN ORAL SLOPE FACTORS FOR ENVIRONMENTAL PCBs | |||
---|---|---|---|
Media | High | Low | Lowest |
Food | Yes | No | No |
Soil | Yes | No | No |
Sediment | Yes | No | No |
Tapwater | No | Yes | No |
Surface Water | No | Yes | No |
Air | Yes | Yes | Yes |
The IRIS Profile offers various criteria for the assignment of Aroclors into risk/persistence tiers. When congeners with more than four chlorines comprise less than one-half of a percent of total PCBs, the lowest risk/persistence tier is appropriate. Aroclor 1016 has virtually no congeners with more than four chlorines and is assigned to the lowest risk/persistence tier (EPA 1996). (Chlorination data and other physicochemical parameters can be found in section 4 of the ATSDR Profile.) Most of the criteria for assignment to the high risk/persistence tier are met by the other Aroclors on the RSL Table as they are typically assessed at environmental sites. In addition to the degree of chlorination, these criteria include: food chain exposure; sediment or soil ingestion; dust or aerosol inhalation; dermal exposure; presence of dioxin-like, tumor-promoting, or persistent congeners; and early-life exposure (all pathways and mixtures). These are default recommendations for purposes of RSL screening. Below represents the assignment of Aroclors to risk/persistence tiers for the RSL tables.
TIERS OF HUMAN ORAL SLOPE FACTORS FOR ENVIRONMENTAL PCBs | |||
---|---|---|---|
Aroclor | High | Low | Lowest |
1016 | No | No | Yes |
1221 | Yes | No | No |
1232 | Yes | No | No |
1242 | Yes | No | No |
1248 | Yes | No | No |
1254 | Yes | No | No |
1260 | Yes | No | No |
Aroclor 5460 toxicity is determined in a PPRTV independently from the IRIS assessment.
If risk assessors believe that the form of PCB and the environmental route at a specific site warrant a different assignment of risk/persistence category, they may consult with regional risk assessors on site-specific cases.
5.9 Xylenes
The IRIS oral RfD of 2E-01 mg/kg-day for xylene, mixture is used as a surrogate for the 3 xylene congeners. The earlier RfD values for some xylene isomers were withdrawn from our electronic version of HEAST. Also, the IRIS inhalation RfC of 1E-01 mg/m3 for xylene, mixture is used as a surrogate for the 3 xylene congeners.
5.10 Arsenic
Arsenic screening levels for ingestion of soil and sediment are now calculated with the default relative bioavailability factor (RBA) of 0.6. The RBA can be adjusted using the calculator in site-specific/user-provided mode the same way toxicity values can be changed. The RBA for soil ingestion is shown in the calculator output. The 2012 document, Compilation and Review of Data on Relative Bioavailability of Arsenic in Soil (PDF) (58 pp, 474 K) provides supporting information.
In 2017, the EPA has released a standard operating procedure for an in vitro bioaccessibility assay for arsenic in soil. The in vitro method for predicting oral RBA of arsenic in soil (EPA SW846 Method 1340) has been validated, and it is now recommended that the in vitro method be used to estimate site-specific RBA, when site-specific RBA is needed. This method can provide a more comprehensive characterization of RBA variability at the site. The default value represents the 95th percentile of many arsenic soil samples, and it is expected that the site-specific RBA will be less than 0.6 at most sites, which means that the default should be protective for screening. This method may also be used for sediments where the incidental ingestion of sediments is being assessed. Site-specific RBAs derived with the in vitro method should be verified with your Regional Risk Assessor. Guidance related to these topics can be found in the Soil Bioavailability at Superfund Sites: Guidance.
Absolute bioavailability can be thought of as the absorption fraction (PDF) (20 pp, 133 K). Relative bioavailability accounts for differences in the bioavailability of a contaminant between the medium of exposure (e.g., soil) and the media associated with the toxicity value (e.g., the arsenic RfD and SFO are derived from drinking water studies). The 60% oral RBA for arsenic in soil is empirically-based. It represents an upper-bound estimate from numerous studies where the oral RBA of soil-borne arsenic in samples collected from across the U.S. was experimentally determined against the water-soluble form. This RBA does not apply to dermal exposures to arsenic in soil for which the absorbed dose is calculated using a dermal absorption fraction (ABSd) of 0.03 (Exhibit 3-4 of USEPA, 2004).
5.11 Total Petroleum Hydrocarbons (TPHs)
Traditionally, hydrocarbon-impacted soils at sites contaminated by releases of petroleum fuels have been managed based on their total petroleum hydrocarbon (TPH) content. TPH refers to the total mass of hydrocarbons present without identifying individual compounds. In practice, TPH is defined by the analytical method that is used to measure the hydrocarbon content in contaminated media. Since the hydrocarbon extraction efficiency is not identical for each method, the same sample analyzed by different TPH methods will produce different TPH concentrations.
The hazard and health risk assessments that are typically conducted to support risk management decisions at contaminated sites generally require some level of understanding of the hydrocarbon chemical composition present in the contaminated media. Traditional TPH measurement techniques, however, provide no specific information about the detected hydrocarbons. Because TPH is not a consistent entity, the assessment of health effects and development of toxicity values for mixtures of hydrocarbons are problematic. In fact, many risk assessors prefer to analyze and assess the individual chemical constituents rather than rely on TPH data; consult with your regional risk assessor for site-specific recommendations. In cases where TPH data are used, more details about the provisional TPH toxicity values are provided below.
In 2022, the Superfund Health Risk Technical Support Center National Center for Environmental Assessment Office of Research and Development published a document that provides the data, methods, and assumptions for deriving Provisional Peer-Reviewed Toxicity Values (PPRTVs) for six fractions of petroleum hydrocarbons. The six TPH fractions were assigned representative compounds for determination of toxicity values and chemical-specific parameters to calculate RSLs.
The PPRTV paper was the principal source for the derivation of these values. The carbon ranges and representative compounds for the RfDs, RfCs, and chemical-specific parameters are listed in the table below. An average of the chemical-specific parameters for 1,3,5-trimethylbenzene, 1,2,4-trimethylbenzene, and 1,2,3-trimethylbenzene was calculated for the medium aromatic fraction. This is because all three are representative compounds for chronic and subchronic RfDs and RfCs. An average of the chemical-specific parameters for n-heptane, n-hexane, and cyclohexene was calculated for the low aliphatic fraction. This is because n-heptane represents the chronic RfC, n-hexane represents the subchronic RfC, and cyclohexene represents the the chronic and subchronic RfDs.
The carbon ranges, presented in the PPRTV assessment, may not exactly match the carbon ranges from laboratory results for TPH fractions. Additionally, the carbon ranges, presented in the PPPRTV assessment, may not match laboratory results for DRO, GRO, ORO, or RRO analysis. The TPH carbon ranges used in the RSLs are not intended to screen against DRO, GRO, ORO and RRO analysis. While the carbon ranges for TPHs and range organics may not exactly match, with direction from your regional risk assessor, reasonable assignment may be achieved.
TPH Fractions | Number of Carbons | Equivalent Carbon Number Index |
Representative |
Representative |
---|---|---|---|---|
Low aliphatic | C5-C8 | EC5-EC8 | cyclohexene/n-heptane and n-hexane | average of cyclohexene, n-heptane, and n-hexane |
Medium aliphatic | C9-C18 | EC>8-EC16 | midrange aliphatic hydrocarbon streams | n-nonane* |
High aliphatic | C19-C32 | EC>16-EC35 | white mineral oil | white mineral oil |
Low aromatic | C6-C8 | EC6-EC<9 | benzene | benzene |
Medium aromatic | C9-C10 | EC9-EC<11 | 1,3,5-trimethylbenzene, 1,2,4-trimethylbenzene, and 1,2,3-trimethylbenzene | average of 1,3,5-trimethylbenzene, 1,2,4-trimethylbenzene, and 1,2,3-trimethylbenzene |
High aromatic | C10-C32 | EC>11-EC35 | benzo[a]pyrene | benzo[a]pyrene |
*A medium aliphatic representative compound was not listed in the PPRTV paper so n-nonane was selected by the RSL work-group to represent the chemical-specific parameters.
Within the Superfund program, when TPHs are considered in the site characterization, they are assessed in supplemental health risk assessments only for potential noncancer health effects. Therefore, starting with the May 2014 update the RSLs are no longer calculated using cancer toxicity values. Most of the carcinogens in the TPH carbon range are individually listed on the RSL table. Combining TPH and individual constituent cancer risks would be overly protective.
5.12 Soil Saturation Limit (Csat)
The soil saturation concentration, Csat, corresponds to the contaminant concentration in soil at which the absorptive limits of the soil particles, the solubility limits of the soil pore water, and saturation of soil pore air have been reached. Above this concentration, the soil contaminant may be present in free phase (i.e., nonaqueous phase liquids (NAPLs) for contaminants that are liquid at ambient soil temperatures and pure solid phases for compounds that are solid at ambient soil temperatures). Csat is not calculated for chemicals that are solid at ambient soil temperatures. The following decision criteria was established from SSL guidance, Table C-3: if melting point is less than 20 °C, chemical is a liquid; if melting point is above 20 °C, chemical is solid.
Equation 4-10 is used to calculate Csat for each volatile contaminant. As an update to RAGS HHEM, Part B (USEPA 1991a), this equation takes into account the amount of contaminant that is in the vapor phase in soil in addition to the amount dissolved in the soil's pore water and sorbed to soil particles. If H' is not available, Csat can still be calculated.
Chemical-specific Csat concentrations must be compared with each VF-based inhalation SL because a basic principle of the SL volatilization model is not applicable when free-phase contaminants are present. How these cases are handled depends on whether the contaminant is liquid or solid at ambient temperatures. Liquid contaminants that have a VF-based inhalation SL that exceeds the Csat concentration are set equal to Csat. For organic compounds that are solids (e.g., PAHs), soil screening decisions are based on the appropriate SLs for other pathways of concern at the site (e.g., ingestion). Note, that the SLs presented for soil inhalation in the RSL tool combine the VF and the PEF components. If the Csat substitution is performed, the whole SL is replaced and not just the VF component.
The RSL tables and the default calculator settings do not substitute Csat for risk-based calculations. If the risk-based concentration exceeds Csat, the resulting SSL concentration may be overly protective. This is because the dissolved, absorbed and vapor concentrations cease to rise linearly as soil concentration increases above the Csat level (pure product or nonaqueous phase liquid (NAPL) is present). The SSL model used in the RSL calculator is not a four phase model. If a NAPL is present at your site more sophisticated models may be necessary. The calculator, if operated in site-specific mode, will give the option to apply the Csat substitution rule. H' changes when groundwater temperature changes. Please see section 4.9.9 of this user guide for H' determination at temperature other than 25°C.
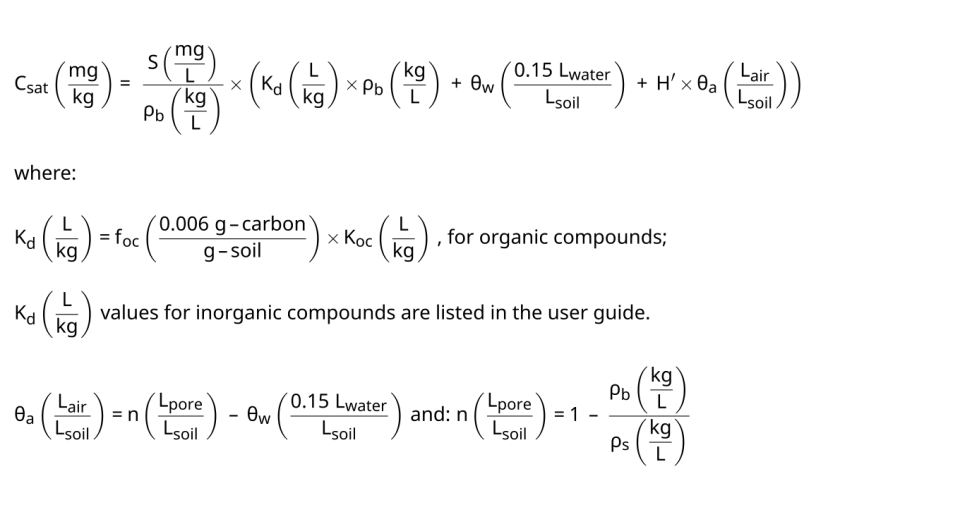
5.13 SL Theoretical Ceiling Limit
The ceiling limit of 10+5 mg/kg is equivalent to a chemical representing 10% by weight of the soil sample. At this contaminant concentration (and higher), the assumptions for soil contact may be violated (for example, soil adherence and wind-borne dispersion assumptions) due to the presence of the foreign substance itself.
The RSL tables and the default calculator settings do not substitute the theoretical ceiling limit for risk-based calculations but they do indicate if the resulting RSL has exceeded the theoretical ceiling limit in the key. The calculator, if operated in site-specific mode, will give the option to apply the theoretical ceiling limit.
5.14 Target Risk
With the exceptions described previously, SLs are chemical concentrations that correspond to fixed levels of risk (i.e., either a one-in-one million [10-6] for cancer risk or a noncarcinogenic hazard quotient of 1) in soil, air, and water. In noncarcinogenic equations, THQ represents the target hazard quotient and is used for individual substances or exposure routes like: ingestion, dermal, and inhalation. The target hazard index (THI), is the target across multiple substances or exposure routes. In most cases, where a substance causes both cancer and noncancer (systemic) effects, the 10-6 cancer risk will result in a more stringent criteria and consequently this value is presented in the printed copy of the Table. SL concentrations that equate to a 10-6 cancer risk are indicated by 'ca' in the calculator and 'c' in the generic tables. SL concentrations that equate to a hazard quotient (HQ) of 1 for noncarcinogenic concerns are indicated by 'nc' in the calculator and 'n' in the generic tables.
If the SLs are to be used for site screening, it is recommended that both cancer and noncancer-based SLs be used. Both carcinogenic and noncarcinogenic values may be obtained in the Supporting Tables.
Some users of this SL Table may plan to multiply the cancer SL concentrations by 10 or 100 to set 'action levels' for triggering remediation or to set less stringent cleanup levels for a specific site after considering non-risk-based factors such as ambient levels, detection limits, or technological feasibility. This risk management practice recognizes that there may be a range of values that may be 'acceptable' for carcinogenic risk (EPA's risk management range is one-in-a-million [10-6] to one-in-ten thousand [10-4]). However, this practice could lead one to overlook serious noncancer health threats and it is strongly recommended that the user consult with a toxicologist or regional risk assessor before doing this. Carcinogens are indicated by an asterisk ('*') in the SL Table where the noncancer SLs would be exceeded if the cancer value that is displayed is multiplied by 100. ('**') indicate that the noncancer values would be exceeded if the cancer SL were multiplied by 10. There is no range of 'acceptable' noncarcinogenic 'risk' for CERCLA sites. Therefore, the noncancer SLs should not be multiplied by 10 or 100 when setting final cleanup criteria. In the rare case where noncancer SLs are more stringent than cancer SLs set at one-in-one-million risk, a similar approach has been applied (e.g. 'max').
SL concentrations in the printed Table are risk-based, but for soil there are two important exceptions: (1) for several volatile chemicals, SLs may exceed the soil saturation level ('sat') and (2) SLs may exceed a non-risk based 'ceiling limit' concentration of 10+5 mg/kg ('max') for relatively less toxic inorganic and semivolatile contaminants. For more information on the 'sat' value in the SL Table, please see the discussion in Section 5.11. For more information on the 'max' value in the SL Table, please see the discussion in Section 5.13.
With respect to applying a 'ceiling limit' for chemicals other than volatiles, it is recognized that this is not a universally accepted approach. Some within the agency argue that all values should be risk-based to allow for scaling (for example, if the risk-based SL is set at a hazard quotient = 1.0, and the user would like to set the hazard quotient to 0.1 to take into account multiple chemicals, then this is as simple as multiplying the risk-based SL by 1/10th). If scaling is necessary, SL users can do this simply by referring to the Supporting Tables at this website where risk-based soil concentrations are presented for all chemicals.
In spite of the fact that applying a ceiling limit is not a universally accepted approach, this table applies a 'max' soil concentration to the SL Table for the following reasons:
- Risk-based SLs for some chemicals in soil exceed unity (>1,000,000 mg/kg), which is not possible.
- The ceiling limit of 10+5 mg/kg is equivalent to a chemical representing 10% by weight of the soil sample. At this contaminant concentration (and higher), the assumptions for soil contact may be violated (for example, soil adherence and wind-borne dispersion assumptions) due to the presence of the foreign substance itself.
- SLs currently do not address short-term exposures (e.g., pica children and construction workers). Although extremely high soil SLs are likely to represent relatively non-toxic chemicals, such high values may not be justified if in fact more toxicological data were available for evaluating short-term and/or acute exposures.
5.15 Screening Sites with Multiple Contaminants
Users needing to screen sites with multiple contaminants should consult with their regional risk assessors. See the Frequent Questions page for information on use of the THQ=1.0 versus the THQ=0.1 tables.
5.16 Deriving Soil Gas SLs
The air SLs could apply to indoor air from, e.g., a vapor intrusion scenario. To model indoor air concentrations from other media (e.g., soil gas, groundwater), consult with regional experts in vapor intrusion.
For more information on EPA's current understanding of this emerging exposure pathway, please refer to EPA's recent draft guidance Evaluating the Vapor Intrusion to Indoor Air Pathway from Groundwater and Soils (Subsurface Vapor Intrusion Guidance) (USEPA 2002).
5.17 Mutagens
Some of the carcinogens in this tool operate by a mutagenic mode of action for carcinogenesis. It's likely that some chemicals with a mutagenic mode of action, which would be expected to cause irreversible changes to DNA, would exhibit a greater effect in early-life versus later-life exposure. Cancer risk to children in the context of the U.S. Environmental Protection Agency's cancer guidelines (U.S. EPA, 2005 (PDF) (166 pp, 468 K)) includes both early-life exposures that may result in the occurrence of cancer during childhood and early-life exposures that may contribute to cancers later in life. In keeping with this guidance, separate cancer risk equations are presented for mutagens. The mutagen vinyl chloride has a unique set of equations. The IRIS profile should be consulted for the intent of non-prorated early life exposure combined with prorating continuous life exposure. Consult Supplemental Guidance for Assessing Susceptibility from Early-Life Exposure to Carcinogens, EPA/630/R-03/003F, March 2005 (PDF) (126 pp, 1.78 MB) for further information on mutagens.
The below table lists the chemicals considered to be carcinogenic by mutagenic mode of action for the purposes of the RSLs. Also provided in the table is a link to the source as to why the chemical is considered to be a mutagen.
Chemical | CASRN | Reference |
---|---|---|
Acrylamide | 79-06-1 | IRIS |
Benz[a]anthracene | 56-55-3 | Benzo[a]pyrene* |
Benzidine | 92-87-5 | Supplemental Guidance |
Benzo[a]pyrene | 50-32-8 | Supplemental Guidance |
Benzo[b]fluoranthene | 205-99-2 | Benzo[a]pyrene* |
Benzo[k]fluoranthene | 207-08-9 | Benzo[a]pyrene* |
Chloroprene | 126-99-8 | IRIS |
Chromium(VI) | 18540-29-9 | IRIS |
Chrysene | 218-01-9 | Benzo[a]pyrene* |
Coke Oven Emissions | 8007-45-2 | 70 Federal Register 19992 |
Dibenz[a,h]anthracene | 53-70-3 | Supplemental Guidance |
Dibromo-3-chloropropane, 1,2- | 96-12-8 | PPRTV |
Dimethylbenz(a)anthracene, 7,12- | 57-97-6 | Supplemental Guidance |
Dimethylbenzidine, 3,3' | 119-93-7 | PPRTV |
Dimethoxybenzidine, 3,3'- | 119-90-4 | PPRTV |
Ethylene Oxide | 75-21-8 | IRIS |
Formaldehyde | 50-00-0 | IRIS |
Indeno[1,2,3-cd]pyrene | 193-39-5 | Benzo[a]pyrene* |
Methylcholanthrene, 3- | 56-49-5 | Supplemental Guidance |
Methylene Chloride | 75-09-2 | IRIS |
Methylene-bis(2-chloroaniline), 4,4'- | 101-14-4 | PPRTV |
Nitrosodiethylamine, N- | 55-18-5 | Supplemental Guidance |
Nitrosodimethylamine, N- | 62-75-9 | Supplemental Guidance |
Nitroso-N-ethylurea, N- | 759-73-9 | Supplemental Guidance |
Nitroso-N-methylurea, N- | 684-93-5 | Supplemental Guidance |
Phenylenediamine, o- | 95-54-5 | PPRTV |
Safrole | 94-59-7 | Supplemental Guidance |
Trichloroethylene | 79-01-6 | IRIS |
Trichloropropane, 1,2,3- | 96-18-4 | IRIS |
Urethane | 51-79-6 | Supplemental Guidance |
Vinyl Chloride | 75-01-4 | Supplemental Guidance |
* Please see section 2.3.6 of this user guide regarding Relative Potency Factors (RPFs).
5.18 Trichloroethylene (TCE)
It is recommended that a regional risk assessor be consulted when evaluating TCE in any medium especially when less than chronic exposure scenarios are considered. The Superfund program issued a Compilation of Information Relating of Early/Interim Actions at Superfund Sites and the TCE IRIS Assessment (PDF) (3 pp, 929 K) memo in August 2014. Several regions have issued their own guidance as well.
In order to make the calculator display the correct results for TCE, the standard cancer and mutagen equations needed to be combined. Since TCE requires the use of different toxicity values for cancer and mutagen equations, it was decided to make a toxicity value adjustment factor for cancer (CAF) and mutagens (MAF). The adjustments were done for oral (o) and inhalation (i). These adjustment factors are used in the TCE equation images presented in section 4. The equations used are presented below. The adjustment factors are based on the adult-based toxicity values and these are the cancer toxicity values presented in the Generic Tables.
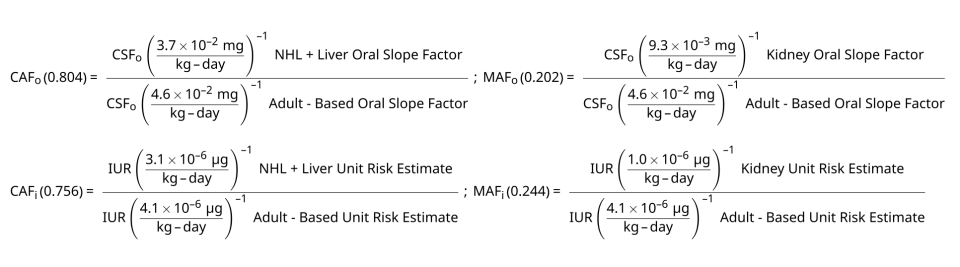
5.19 Mercuric Chloride (and other Mercury salts)
The IRIS RfC for "Mercury (elemental)" is used as a surrogate for "Mercuric Chloride (and other Mercury salts)". Note, that the VF for "Mercury (elemental)" is not used as a surrogate for "Mercuric Chloride (and other Mercury salts)". The use of the surrogate RfC would appear to be a violation of the RSL toxicity hierarchy because Cal EPA offers a RfC for Mercuric Chloride. However, the actual form of mercury evaluated for the Cal EPA RfC was elemental mercury. Since IRIS already had a RfC for "Mercury (elemental)", it was decided to use the tier 1 source over a tier 3 source.
5.20 Cyanide (CN-)
The IRIS RfC for "Hydrogen Cyanide" is used as a surrogate for "Cyanide (CN-)".
5.21 Thallic Oxide and Thallium Selenite
The oral RfD for thallic oxide, used in this website, is derived from the PPRTV oral RfD for thallium sulfate by molecular weight (MW) adjustments and stoichiometric calculations. Thallic oxide (Tl2O3) has a MW of 456.765 and thallium sulfate (Tl2SO4) has a MW of 504.82. To derive the oral RfD of 2E-05 mg/kg-day for thallic oxide, the thallium sulfate RfD of 2E-05 mg/kg-day is multiplied by the MW of thallic oxide (456.765) divided by the MW of thallium sulfate (504.82). The oral RfD for thallium selenite, used in this website, is derived from the PPRTV oral RfD for thallium by molecular weight (MW) adjustments and stoichiometric calculations. Thallium selenite (TlSe) has a MW of 283.34 and thallium (Tl) has a MW of 204.38. To derive the oral RfD of 1E-05 mg/kg-day for thallium selenite, the thallium RfD of 1E-05 mg/kg-day is multiplied by the MW of thallium selenite (283.34) divided by the MW of thallium (204.38).
5.22 Polycyclic Aromatic Hydrocarbons (PAHs)
For PAHs in soil, we have not made any recommendations on a default value, which is to say that the default assumption remains that these are 100% bioavailable. There is also no available in vitro method to estimate the oral RBA of PAHs. A small number of sites have elected to run swine or rat models to assess oral RBA, and the TRW has reviewed them before the RBA was accepted for use at the site. Guidance related to these topics can be found in the Soil Bioavailability at Superfund Sites Guidance.
5.23 Refractory Ceramic Fibers (units in fibers)
The ATSDR chronic RfC for refractory ceramic fibers is presented in units of fibers/cm3 (0.03 fibers/cm3). The RfC presented in the RSL tables and calculator is converted to units of fibers/m3 (3E+04 fibers/m3), to be consistent with all other chemicals where the RfC unit is mg/m3. When the chronic RfC is used in the standard RSL air inhalation equations, the resulting units are not in µg/m3 like all the other chemicals, rather the units are in fibers/m3. RSLs are only calculated for air as the medium. The air values in the RSL table are calculated using the equations below to give RSLs in fibers/m3.
Resident Air Noncancer Equation
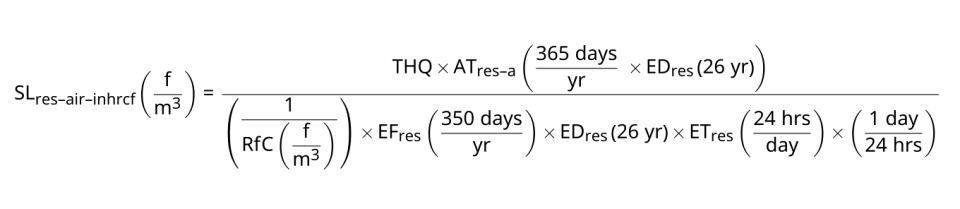
Composite Worker Air Noncancer Equation
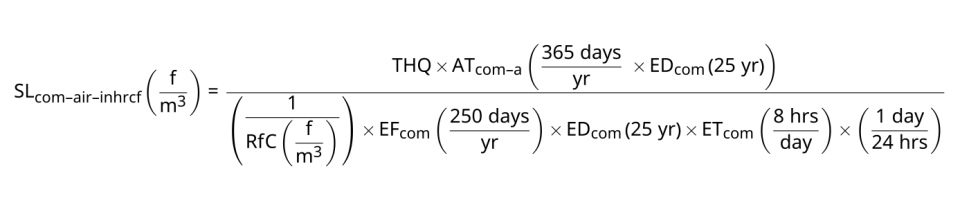
Indoor Worker Air Noncancer Equation
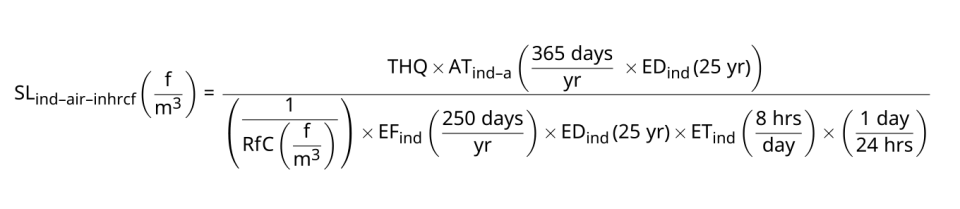
Outdoor Worker Air Noncancer Equation
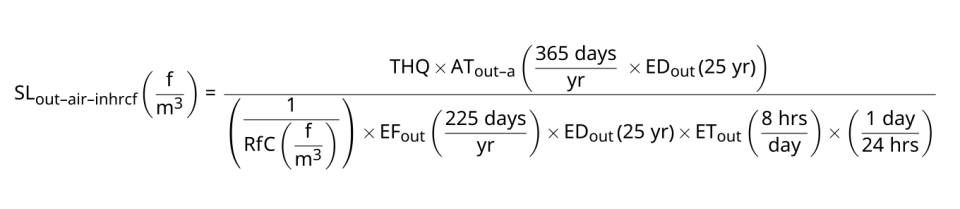
Land Use | THI = 0.1 | THI = 1.0 |
---|---|---|
Resident | 3128 | 31286 |
Composite Worker | 13140 | 131400 |
Indoor Worker | 13140 | 131400 |
Outdoor Worker | 14600 | 146000 |
5.24 Lanthanum Salts
The oral chronic RfDs for lanthanum salts, used in this website, are derived from the PPRTV oral chronic RfD for lanthanum by molecular weight (MW) adjustments and stoichiometric calculations. Lanthanum chloride, anhydrous (LaCl3) has a MW of 245.27, and lanthanum (La) has a MW of 138.91. To derive the chronic oral RfD of 2.83E-05 mg/kg-day for lanthanum chloride, anhydrous, the lanthanum RfD of 5E-05 mg/kg-day is multiplied by the MW of lanthanum (138.91) divided by the MW of lanthanum chloride, anhydrous (245.27). To derive the chronic oral RfD of 1.87E-05 mg/kg-day for lanthanum chloride heptahydrate, the lanthanum RfD of 5E-05 mg/kg-day is multiplied by the MW of lanthanum (138.91) divided by the MW of lanthanum chloride heptahydrate (371.37). To derive the chronic oral RfD of 1.60E-05 mg/kg-day for lanthanum nitrate hexahydrate, the lanthanum RfD of 5E-05 mg/kg-day is multiplied by the MW of lanthanum (138.91) divided by the MW of lanthanum nitrate hexahydrate (433.01). To derive the chronic oral RfD of 2.08E-05 mg/kg-day for lanthanum acetate hydrate, the lanthanum RfD of 5E-05 mg/kg-day is multiplied by the MW of lanthanum (138.91) divided by the MW of lanthanum acetate hydrate (334.05).
5.25 MCLs for Trihalomethanes and Haloacetic Acids
The individual trihalomethanes (bromodichloromethane; bromoform; dibromochloromethane, chloroform) all have the MCL of 80 µg/L listed in the RSL table. However, 80 µg/L is the MCL for Total Trihalomethanes. The individual haloacetic acids (dichloroacetic acid, trichloroacetic acid, chloroacetic acid, bromoacetic acid, and dibromoacetic acid) all have the MCL of 60 µg/L listed in the RSL table. However, 60 µg/L is the MCL for Total haloacetic acids.
5.26 Perchlorate
The tapwater screening level for Perchlorate of 14 µg/L is different from the preliminary remedial goal (PRG) of 15 µg/L calculated by the Office of Solid Waste and Emergency Response in its January 8, 2009, guidance. As described in the OSWER memorandum (PDF), the Agency issued an Interim Drinking Water Health Advisory (Interim Health Advisory) for exposure to perchlorate of 15 µg/L in water. A health advisory provides technical guidance to federal, state, and other public health officials on health effects, analytical methods and treatment technologies associated with drinking water contamination. The Interim Health Advisory for perchlorate was developed using EPA's RfD of 7E-04 mg/kg-day and representative body weight, as well as 90th percentile drinking water and national food exposure data for pregnant women in order to protect the most sensitive population identified by the National Research Council (NRC) (i.e., the fetuses of pregnant women who might have hypothyroidism or iodide deficiency).
The NCP (40 CFR 300.430(e)(2)(A)(1)) provides that when establishing acceptable exposure levels for use as remediation goals (for a Superfund site), consideration must be given to concentration levels to which the human population, including sensitive subgroups, may be exposed without adverse effects over a lifetime or part of a lifetime, incorporating an adequate margin of safety. As a result of the publication of the Interim Health Advisory for perchlorate, OSWER recommends that where no federal or state applicable or relevant and appropriate (ARAR) requirements exist under federal or state laws, 15 µg/L (or 15 ppb) is recommended as the PRG for perchlorate when making CERCLA site-specific cleanup decisions where there is an actual or potential drinking water exposure pathway. However, where State regulations qualify as ARARs for perchlorate, the remediation goals established shall be developed considering the State regulations that qualify as ARARs, as well as other factors cited in the NCP (see 40 CFR 300.430(e)(2)(i)(ff)). Final remediation goals and remedy decisions are made in accordance with 40 CFR300.430 (e) and (f) and associated provisions.
Preliminary remediation goals are the starting points in the development of final cleanup levels at sites. As at all sites addressed under the NCP, these goals may be modified, depending on physical characteristics of a site, State laws and guidance, and other site specific factors, such as additional exposure routes.
The tapwater screening level of 14 µg/L is based on EPA's RfD and using standard RSL equations.
5.27 Styrene-acrylonitrile trimer (SAN Trimer)
Styrene-acrylonitrile trimer (SAN Trimer) is a by-product of specific manufacturing processes for polymers of styrene and acrylonitrile and a mixture of isomers formed by the condensation of two moles of acrylonitrile and one mole of styrene and has a molecular weight of 210. The mixture is composed of two structural forms: 4-cyano-1,2,3,4-tetrahydro-a-methyl1-naphthaleneacetonitrile (THNA, CASRN 57964-39-3) and 4-cyano-1,2,3,4-tetrahydro-1-naphthalenepropionitrile (THNP, CASRN 57964-40-6). The THNA form consists of four stereoisomers: cis-R-THNA (CASRN 142759-38-4), cis-S-THNA (CASRN 142759-39-5), trans-R-THNA (CASRN 142759-37-3), and trans-S-THNA (CASRN 142759-40-8). The THNP form consists of two stereoisomers: cis-THNP (CASRN 142681-91-2) and trans-THNP (CASRN 142681-92-3). The NTP report provides details regarding the structure and toxicity study for the mixture.
The SAN Trimer mixture is analyzed in environmental media using gas chromatography that yields three peaks. The total concentration corresponds to the total area of the three peaks. The Regional Screening Levels provide RSLs based on the oral Reference Dose for the SAN Trimer derived from the toxicity study described above. The PPRTV RfD used to derive the RSLs is based on the SAN Trimer mixture. The SAN Trimer structural isomers are listed separately in the RSL calculator but are the same values for the individual isomers. To evaluate the non-cancer toxicity of the SAN Trimer, compare the total concentration calculated based on the total of the three peaks to the RSL for either isomer.
5.28 cis- and trans-Chlordane Surrogate
According to an April 7, 2021 memo from the Office of Research and Development,
“..technical chlordane can be considered a suitable surrogate for oral, noncancer screening-level assessments of cis- and trans-chlordane”.
Beginning in May 2021, the RSLs will use the technical chlordane (12789-03-6) Reference Dose as a surrogate for cis-chlordane (5103-71-9, alpha-chlordane) and trans-chlordane (5103-74-2, gamma-chlordane).
5.29 Asbestos
Asbestos RSLs are not included in the RSL generic tables, except for the asbestos MCL for resident tap water. Risk estimations are complicated by several factors including unfamiliar exposure units, calculation of exposure point values, as well as different IRIS toxicity factors, and a different risk estimation methodology for general asbestos versus Libby amphibole asbestos. Regional risk assessors are encouraged to consult the Asbestos Technical Review Workgroup (TRW) for assistance by contacting the Asbestos TRW hotline: asbestoshelp@epa.gov. More information is available on the Asbestos TRW website: https://www.epa.gov/superfund/asbestos-superfund-sites-technical-assistance.
5.30 White Phosphorus
IRIS and ATSDR used the name "white phosphorus," with the generic phosphorus CAS of 7723-14-0. Although the RSLs make every effort to retain the chemical name and CAS of the toxicity source, this has presented much confusion. The RSLs assign elemental phosphorus the generic CAS of 7723-14-0 and the allotrope white phosphorus the CAS of 12185-10-3. The toxicity values from IRIS and ATSDR have been assigned to both phosphorus and white phosphorus. Chemical properties for white phosphorus are primarily taken from the ATSDR Toxicity Profile(248 pp, 4.66 MB), but also include other sources.
6. Recommended Default Exposure Parameters
Table 1 presents the definitions of the most commonly used variables and their default values. All the variables and their default values are available in this spreadsheet file or this PDF. The calculator follows the recommendations in the OSWER Directive concerning use of exposure parameters from the 2011 Exposure Factors Handbook. Any alternative values or assumptions used in remedy evaluation or selection on a CERCLA site should be presented with supporting rationale in Administrative Records.
- Table 1. Recommended Default Exposure Parameters and Variable Definitions
Symbol Definition (units) Default Reference SL Screening Level (mg/kg, µg/L, or µg/m3) Contaminant-specific Determined in this calculator TR Target Risk 1 × 10-6 Selected by user THQ Target Hazard Quotient 0.1 Selected by user AT Averaging Time (days) 365 x LT (carcinogenic)
365 x ED (non-carcinogenic)U.S. EPA 1989 (pg. 6-23) LT Lifetime (years) 70 U.S. EPA 1989 (pg. 6-22) IRW Ingestion Rate - Water (L/day) 0.78 (child)
2.5 (adult)OSWER Directive IRS Ingestion Rate - Soil (mg/day) 200 (child)
100 (adult)U.S. EPA 1991a (pg. 15) IRF Ingestion Rate - Fish (mg/day) site-specific Recommend using site-specific values SAres-c Surface Area soil - (cm2/day) 2373 (child - soil)
6032 (adult - soil)
3527 (worker - soil)
6365 (child - water)
19652 (adult - water)OSWER Directive AF Soil Adherence Factor (mg/cm2) 0.2 (child)
0.07 (adult)
0.12 (worker)
0.3 (construction worker)U.S. EPA 2004 (Exhibit 3-5), RAGS Part E BW Body Weight (kg) 15 (child)
80 (adult)U.S. EPA 1991a (pg. 15) ABSd Fraction of contaminant absorbed dermally from soil (unitless) contaminant-specific
Inorganic default = none
VOC default = none
SVOC default = 0.1U.S. EPA 2004 (Exhibit 3-4 and section 3.2.2.4) GIABS Fraction of contaminant absorbed in gastrointestinal tract (unitless) Note: if the GIABS is >50% then it is set to 100% for the calculation of dermal toxicity values. contaminant-specific
inorganic default = 1.0
VOC default = 1.0
SVOC default = 1.0U.S. EPA 2004 (Exhibit 4-1 and section 4.2) EF Exposure Frequency (days/year) 350 (resident)
250 (indoor worker)
225 (outdoor worker)U.S. EPA 1991a (pg. 15) ED Exposure Duration (years) 26 (resident)
6 (child)
20 (adult)
25 (worker)
1 (construction worker)OSWER Directive ET Exposure Time - Air (hours/day) 24 (resident)
8 (worker)The whole day
The work dayETevent Exposure Time - Water (hours/event) 0.54 (child)
0.71 (adult)U.S. EPA 2011, Table 16-28; weighted average of 90th percentile time spent bathing (birth to <6 years) EV Events (events/day) site-specific Site-specific DAF Dilution Attenuation Factor (unitless) 1 (or site-specific) U.S. EPA. 2002 Equation 4-11 PEF Particulate Emission Factor (m3/kg) 1.36 x 109 U.S. EPA 2002 Exhibit D-2 VF Volatilization Factor (m3/kg) contaminant-specific U.S. EPA. 2002 Equation 4-8
7. References
U.S. EPA (Environmental Protection Agency). 1987. Processes, Coefficients, and Models for Simulation Toxic Organics and Heavy Metals in Surface Waters. EPA/600/3-87/015. Office of Research and Development, Athens, GA.
U.S. EPA 1989. Risk assessment guidance for Superfund. Volume I: Human health evaluation manual (Part A)(PDF) (289 pp, 6.9 MB). Interim Final. Office of Emergency and Remedial Response. EPA/540/1-89/002.
U.S. EPA 1991a. Human health evaluation manual, supplemental guidance: "Standard default exposure factors (PDF) (28 pp, 248 K)". OSWER Directive 9285.6-03.
U.S. EPA 1991b. Risk Assessment Guidance for Superfund, Volume I: Human Health Evaluation Manual (Part B, Development of Risk-Based Preliminary Remediation Goals) (PDF) (68 pp, 721 K). Office of Emergency and Remedial Response. EPA/540/R-92/003. December 1991
U.S. EPA. 1996a. Soil Screening Guidance: User's Guide. Office of Emergency and Remedial Response. Washington, DC. OSWER No. 9355.4-23
U.S. EPA. 1996b. Soil Screening Guidance: Technical Background Document. Office of Emergency and Remedial Response. Washington, DC. OSWER No. 9355.4-17A
U.S. EPA. 1997a. Exposure Factors Handbook (PDF) (854 pp, 7.7 MB). Office of Research and Development, Washington, DC. EPA/600/P-95/002Fa.
U.S. EPA 2000. Exposure and Human Health Reassessment of 2,3,7,8-Tetrachlorodibenzo-p-Dioxin (TCDD) and Related Compounds. Part I: Estimating Exposure to Dioxin-Like Compounds. Volume 3--Properties, Environmental Levels, and Background Exposures. Draft Final Report. EPA/600/P- 00/001. Office of Research and Development, Washington, DC. September.
U.S. EPA, 2000b. Methodology for Deriving Ambient Water Quality Criteria for the Protection of Human Health (PDF) (185 pp, 790 K).
U.S. EPA, 2001 (PDF) (38 pp, 185 K). WATER9. Version 1.0.0. Office of Air Quality Planning and Standards, Research Triangle Park, NC. Web site.
U.S. EPA 2002. Supplemental Guidance for Developing Soil Screening Levels for Superfund Sites. OSWER 9355.4-24. December 2002.
U.S. EPA 2004. Risk Assessment Guidance for Superfund Volume I: Human Health Evaluation Manual (Part E, Supplemental Guidance for Dermal Risk Assessment) Final. OSWER 9285.7-02EP. July 2004. Document (PDF) (186 pp, 4.2 MB) and website.
U.S. EPA 2005. Supplemental Guidance for Assessing Susceptibility from Early-Life Exposure to Carcinogens. March 2005. Document (PDF) (166 pp, 468 K)
U.S. EPA 2009. Risk Assessment Guidance for Superfund Volume I: Human Health Evaluation Manual (Part F, Supplemental Guidance for Inhalation Risk Assessment) Final. OSWER 9285.7-82. January 2009. Document (PDF) (68 pp, 724 K), memo (PDF) (3 pp, 487 K) and website.
U.S. EPA 2011. Exposure Factors Handbook 2011 Edition (Final). National Center for Environmental Assessment, Office of Research and Development. Washington D.C. Currently available online at the EFH webpage or download here (PDF) (1436 pp, 21.3 MB).
U.S. EPA 2012. OSWER Directive 9200.1-113: Compilation and Review of Data on Relative Bioavailability of Arsenic in Soil . Washington, DC.
U.S. EPA 2014. OSWER Directive 9200.1-120 (PDF) (7 pp, 1.1 MB). Washington, DC.
U.S. EPA 2019. Exposure Factors Handbook Chapter 3 Updates Office of Research and Development, Washington, DC.
For assistance/questions please use the Regional Screening Levels (RSLs) contact us page. For general risk assessment questions, separate from the RSLs, please use the link below.